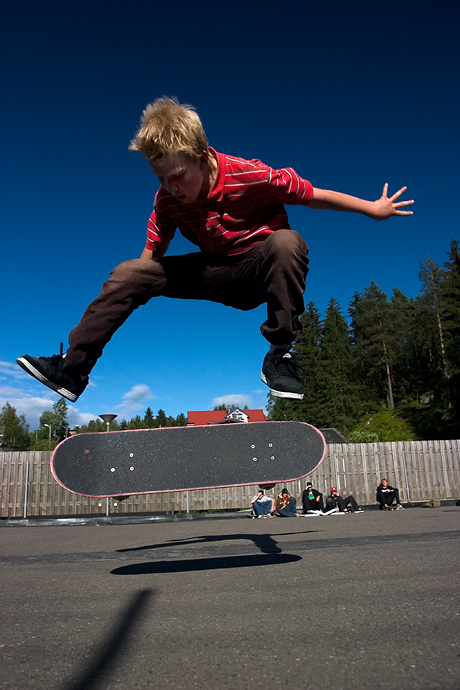
IN THE BLINK OF AN EYE
As you drive into a parking lot, a boy on a skateboard suddenly flies in front of your car across your field of vision. You see the boy in the nick of time and react immediately. You slam on the brakes and steer sharply to the right — all in the blink of an eye. You avoid a collision, but just barely. You’re shaken up, but thankful that no one was hurt. How did you respond so quickly? Rapid responses like this are controlled by your nervous system.
OVERVIEW OF THE NERVOUS SYSTEM
The nervous system, illustrated in the sketch below, is the human organ system that coordinates all of the body’s voluntary and involuntary actions, by transmitting electrical signals to and from different parts of the body. Specifically, the nervous system extracts information from the internal and external environments, using sensory receptors. Usually, it then sends signals encoding this information to the brain, which processes the information to determine an appropriate response. Finally, the brain sends signals to muscles, organs, or glands to bring about the response. In the example above, your eyes detected the boy, the information traveled to your brain, and your brain told your body to act so as to avoid a collision.
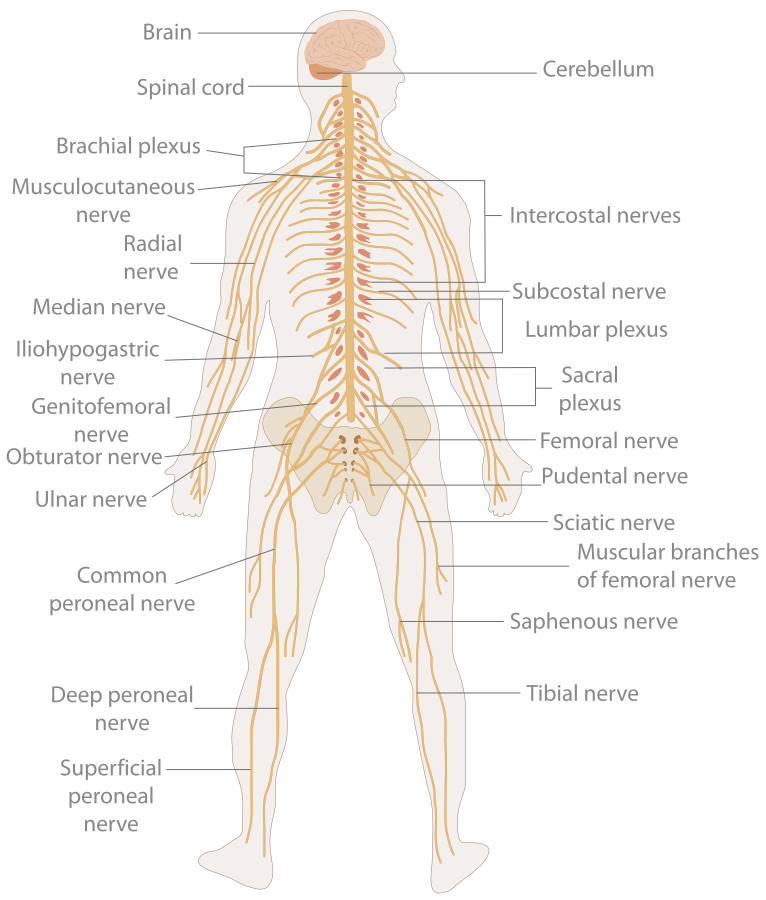
SIGNALS OF THE NERVOUS SYSTEM
The signals sent by the nervous system are electrical signals called nerve impulses, and they are transmitted by special nervous system cells called neurons (or nerve cells), like the one in Figure 8.3. Long projections (called axons) from neurons carry nerve impulses directly to specific target cells. A cell that receives nerve impulses from a neuron (typically a muscle or a gland) may be excited to perform a function, inhibited from carrying out an action, or otherwise controlled. In this way, the information transmitted by the nervous system is specific to particular cells and is transmitted very rapidly. In fact, the fastest nerve impulses travel at speeds greater than 100 metres per second! Compare this to the chemical messages carried by the hormones that are secreted into the blood by endocrine glands. These hormonal messages are “broadcast” to all the cells of the body, and they can travel only as quickly as the blood flows through the cardiovascular system.
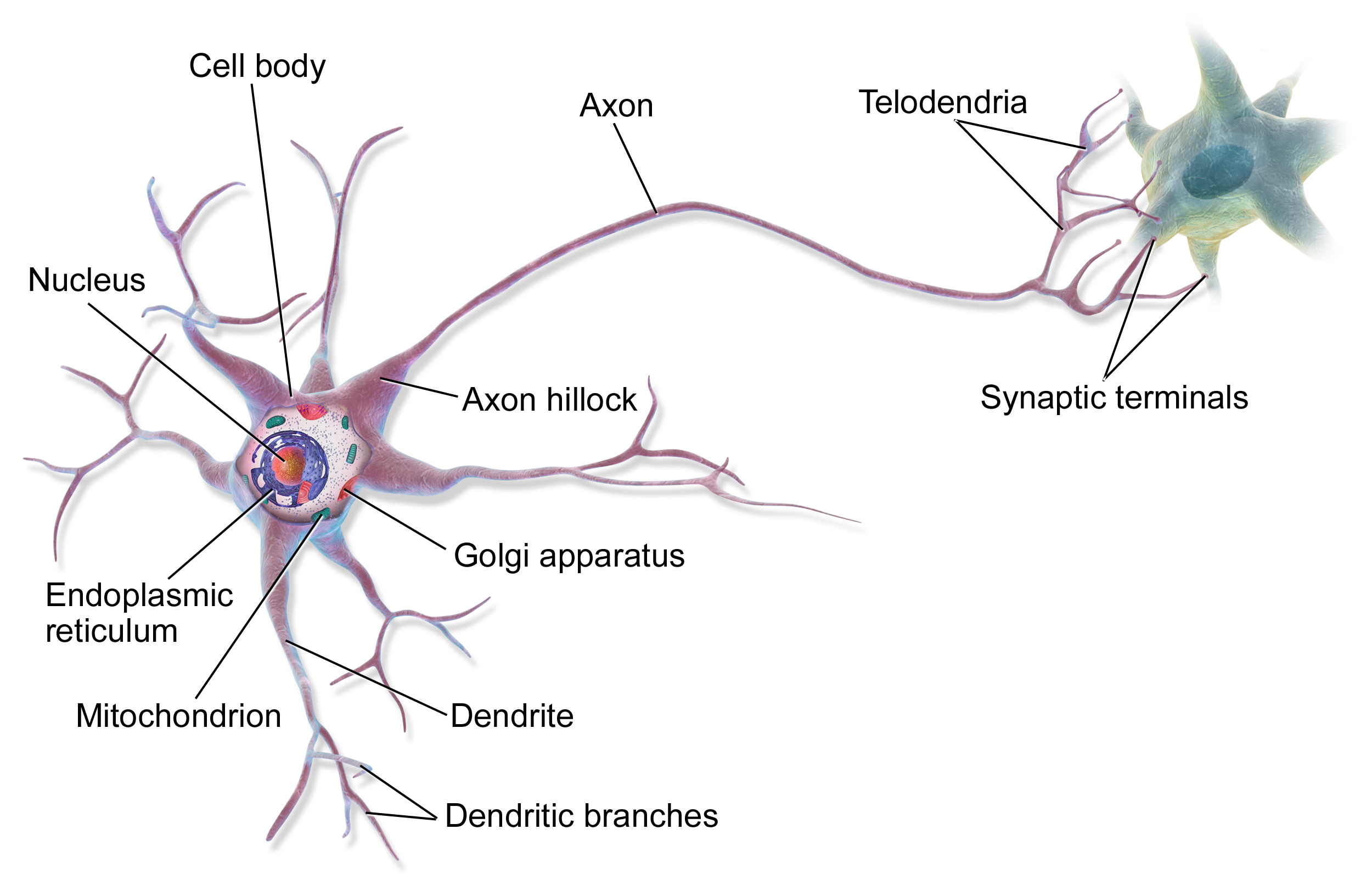
This simple model of a nerve cell shows part of its long axon which carries nerve impulses to other cells. The multiple shorter projections are called dendrites, and they receive nerve impulses from other cells.
ORGANIZATION OF THE NERVOUS SYSTEM
As you might predict, the human nervous system is very complex. It has multiple divisions, beginning with its two main parts, the central nervous system (CNS) and the peripheral nervous system (PNS), as shown in the diagram below (Figure 8.4). The CNS includes the brain and spinal cord, and the PNS consists mainly of nerves, which are bundles of axons from neurons. The nerves of the PNS connect the CNS to the rest of the body.
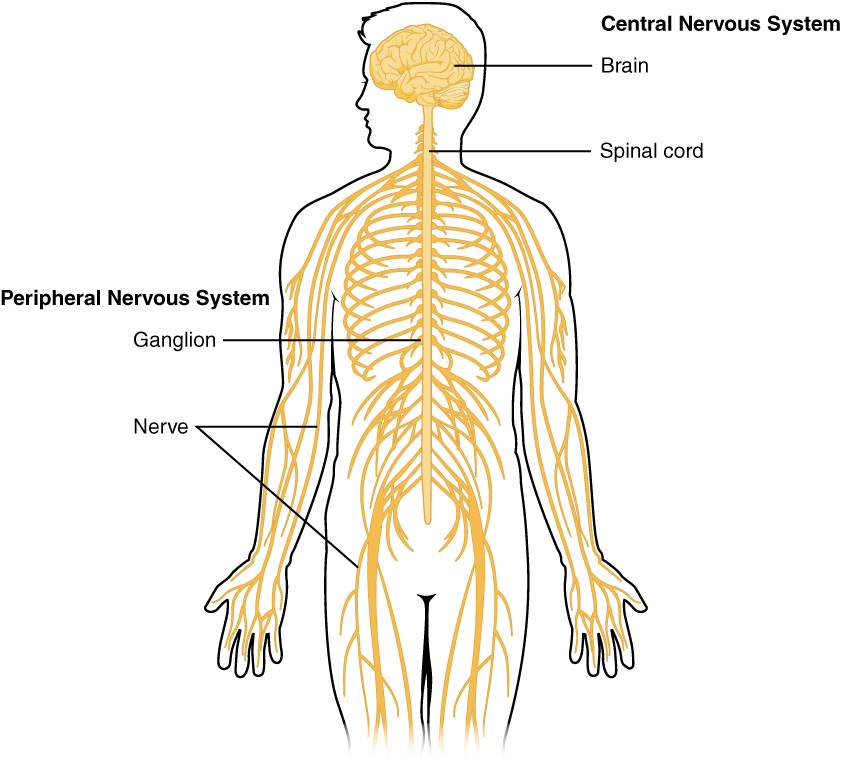
The PNS can be further subdivided into two divisions, known as the autonomic and somatic nervous systems (Figure 8.2.5). These divisions control different types of functions, and they often interact with the CNS to carry out these functions. The somatic nervous system controls activities that are under voluntary control, such as turning a steering wheel. The autonomic nervous system controls activities that are not under voluntary control, such as digesting a meal. The autonomic nervous system has three main divisions: the sympathetic division (which controls the fight-or-flight response during emergencies), the parasympathetic division (which controls the routine “housekeeping” functions of the body at other times), and the enteric division (which provides local control of the digestive system).
Figure 8.5 Divisions of the Nervous System.
Review
- List the general steps through which the nervous system generates an appropriate response to information from the internal and external environments.
- What are neurons?
- Compare and contrast the central and peripheral nervous systems.
- Which major division of the peripheral nervous system allows you to walk to class? Which major division of the peripheral nervous system controls your heart rate?
- Identify the functions of the three main divisions of the autonomic nervous system.
- What is an axon, and what is its function?
- Define nerve impulses.
- Explain generally how the brain and spinal cord can interact with and control the rest of the body.
- How are nerves and neurons related?
- What type of information from the outside environment do you think is detected by sensory receptors in your ears?
8.3 NEURONS AND NEUROGLIA
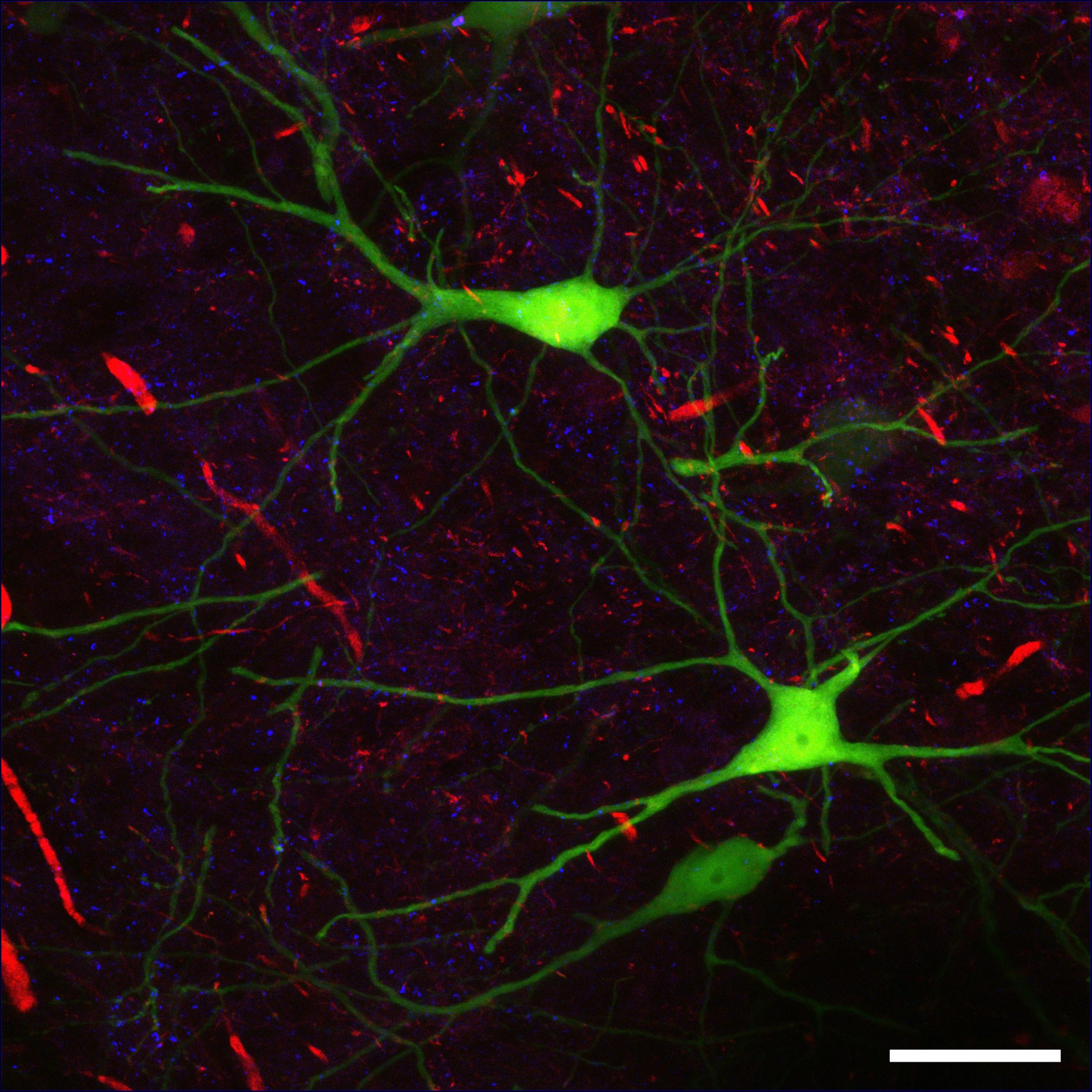
LIFE AS ART
This colorful picture (Figure 8.6) could be an abstract work of modern art. You might imagine it hanging in an art museum or art gallery. In fact, the picture illustrates real life — not artistic creation. It is a micrograph of human nervous tissue. The neon green structures in the picture are neurons. The neuron is one of two basic types of cells in the nervous system. The other type is the neuroglial cell.
Neurons
Neurons – also called nerve cells — are electrically excitable cells that are the main functional units of the nervous system. Their function is to transmit nerve impulses, and they are the only type of human cells that can carry out this function.
NEURON STRUCTURE
Figure 8.7 shows the structure of a typical neuron.
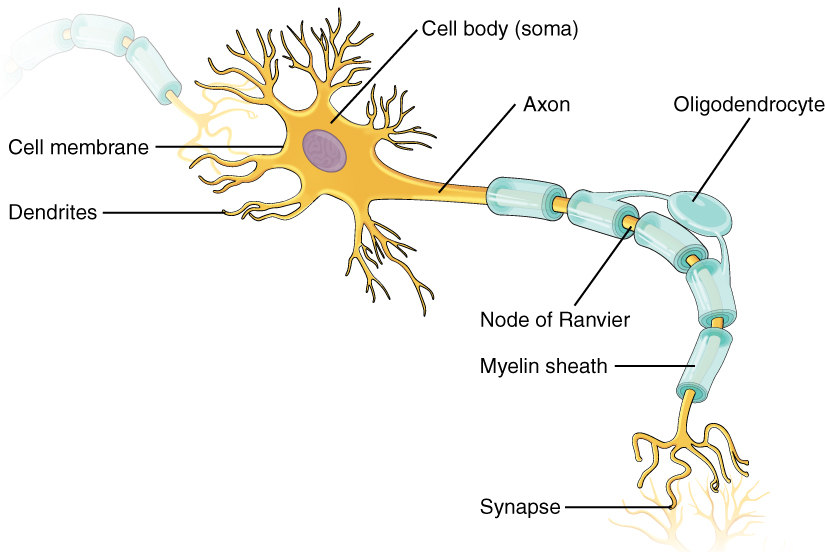
Figure 8.7 The structure of a typical neuron.
NEUROGENESIS
Fully differentiated neurons, with all their special structures, cannot divide and form new daughter neurons. Until recently, scientists thought that new neurons could no longer be formed after the brain developed prenatally. In other words, they thought that people were born with all the brain neurons they would ever have, and as neurons died, they would not be replaced. However, new evidence shows that additional neurons can form in the brain, even in adults, from the division of undifferentiated neural stem cells found throughout the brain. The production of new neurons is called neurogenesis. The extent to which it can occur is not known, but it is not likely to be very great in humans.
NEURONS IN NERVOUS TISSUES
The nervous tissue in the brain and spinal cord consists of gray matter and white matter. Gray matter contains mainly non-myelinated structures, including the cell bodies and dendrites of neurons. It is gray only in cadavers. Living gray matter is actually pinker than gray (see Figure 8.8). White matter consists mainly of axons covered with a myelin sheath, which gives them their white color. White matter also makes up the nerves of the peripheral nervous system. Nerves consist of long bundles of myelinated axons that extend to muscles, organs, or glands throughout the body. The axons in each nerve are bundled together like wires in a cable. Axons in nerves may be more than a meter long in an adult. The longest nerve runs from the base of the spine to the toes.
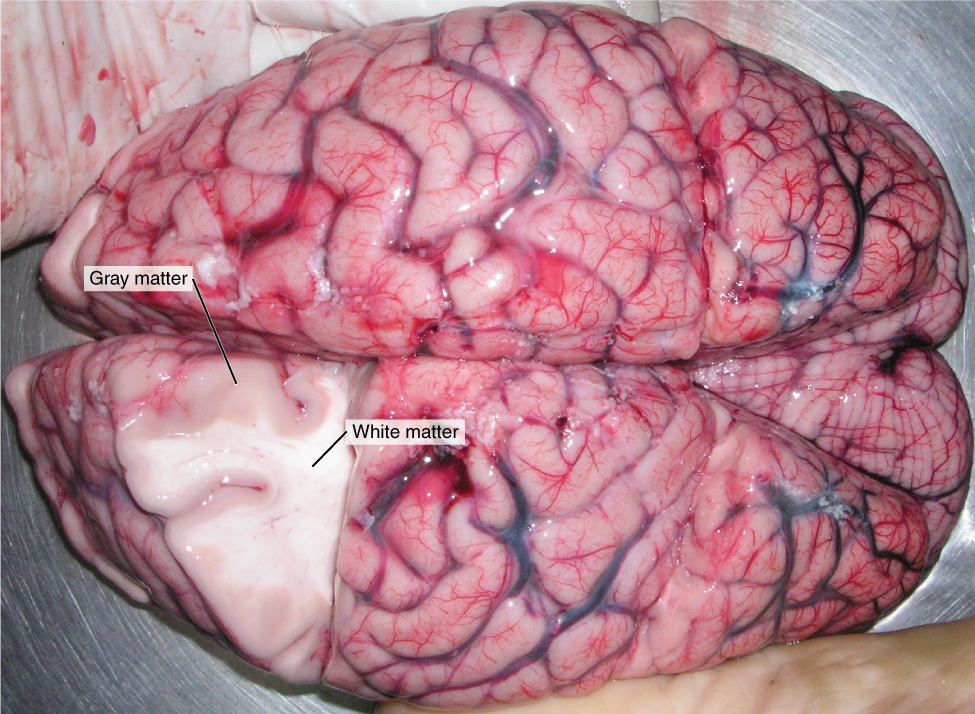
TYPES OF NEURONS
There are hundreds of different types of neurons in the human nervous system that exhibit a variety of structures and functions. Nonetheless, many neurons can be classified functionally based on the direction in which they carry nerve impulses.
- Sensory (also called afferent) neurons carry nerve impulses from sensory receptors in tissues and organs to the central nervous system. They change physical stimuli (such as touch, light, and sound) into nerve impulses.
- Motor (also called efferent) neurons like the one in the diagram below (Figure 8.9), carry nerve impulses from the central nervous system to muscles and glands. They change nerve signals into the activation of these structures.
- Within the spinal cord or brain, interneurons carry nerve impulses back and forth, often between sensory and motor neurons.
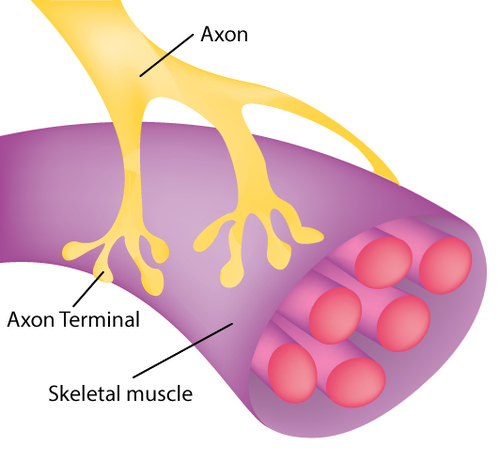
Neuroglia
In addition to neurons, nervous tissues also consist of neuroglia, also called glial cells. The root of the word glial comes from a Greek word meaning “glue,” which reflects earlier ideas about the role of neuroglia in nervous tissues. Neuroglia were thought to be little more than “glue” holding together the all-important neurons, but this is no longer the case. They are now known to play many vital roles in the nervous system. There are several different types of neuroglia, each with a different function. You can see six types of neuroglia in Figure 8.10.
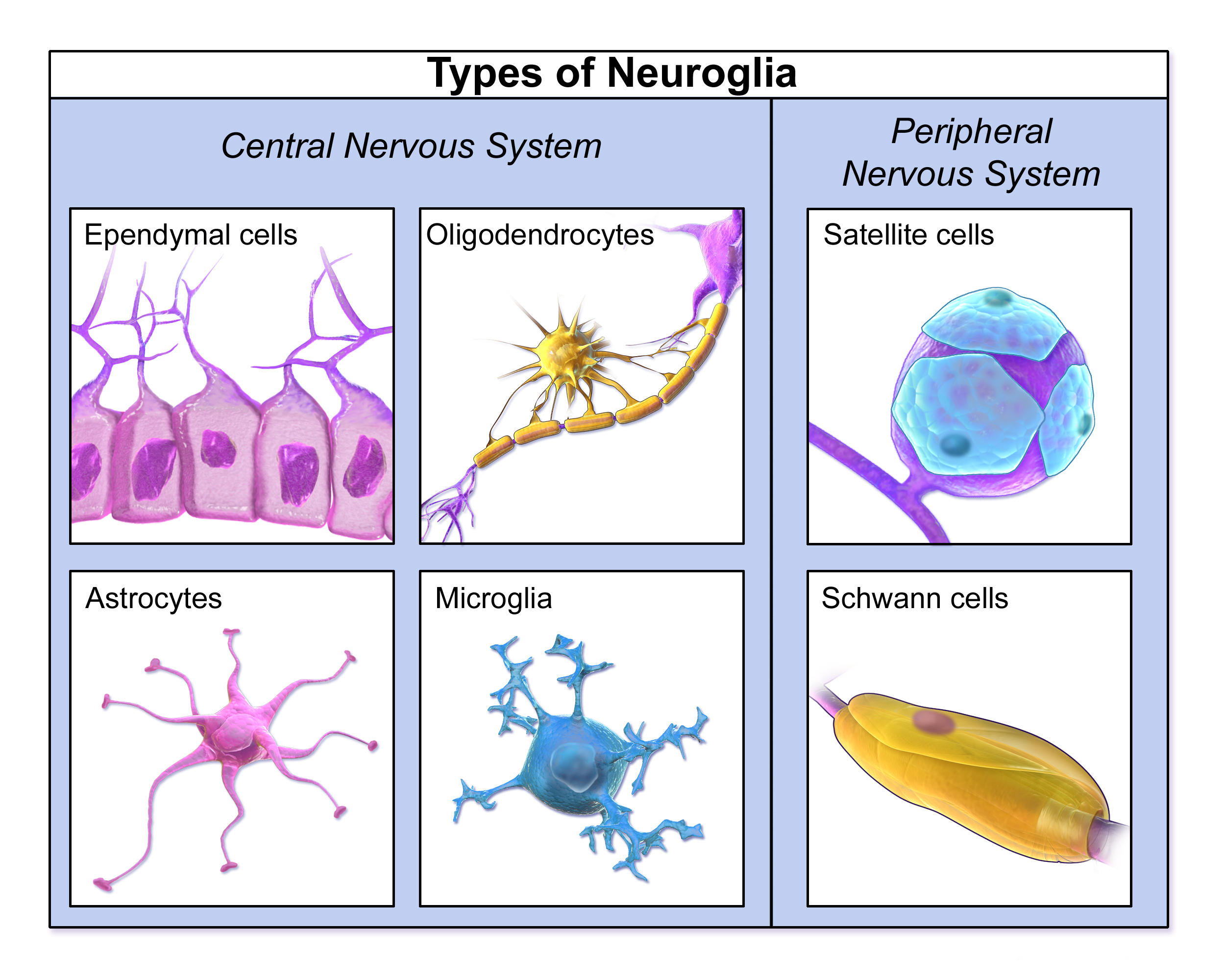
In general, neuroglia provide support for neurons and help them carry out the basic function of nervous tissues, which is to transmit nerve impulses. For example, oligodendrocytes in the central nervous system and Schwann cells in the peripheral nervous system generate the lipids that make up myelin sheaths, which increase the speed of nerve impulses’ transmission. Functions of other neuroglia cells include holding neurons in place, supplying neurons with nutrients, regulating the repair of neurons, destroying pathogens, removing dead neurons, and directing axons to their targets. Neuroglia may also play a role in the transmission of nerve impulses, but this is still under study. Unlike mature neurons, mature glial cells retain the ability to divide by undergoing mitosis.
In the human brain, there are generally roughly equal numbers of neurons and neuroglia. If you think intelligence depends on how many neurons you have, think again. Having a relatively high number of neuroglia is actually associated with higher intelligence. When Einstein’s brain was analyzed, researchers discovered a significantly higher-than-normal ratio of neuroglia to neurons in areas of the brain associated with mathematical processing and language. On an evolutionary scale, as well, an increase in the ratio of neuroglia to neurons is associated with greater intelligence in species.
FEATURE: MY HUMAN BODY
Would you like your brain to make new neurons that could help you become a better learner? When it comes to learning new things, what college student wouldn’t want a little more brain power? If research about rats applies to humans, then sustained aerobic exercise (such as running) can increase neurogenesis in the adult brain, and specifically in the hippocampus, a brain structure important for learning temporally and/or spatially complex tasks, as well as memory. Although the research is still at the beginning stages, it suggests that exercise may actually lead to a “smarter” brain. Even if the research results are not ultimately confirmed for humans, though, it can’t hurt to get more aerobic exercise. It is certainly beneficial for your body, if not your brain!
Review
- Be able to distinguish between the terms Dendrites, Axons, Cell Body, Myelin Sheath.
- Describe the myelin sheath and nodes of Ranvier. How does their arrangement allow nerve impulses to travel very rapidly along axons?
- Define neurogenesis. What is the potential for neurogenesis in the human brain?
- Relate neurons to different types of nervous tissues.
- Compare and contrast sensory and motor neurons.
- Identify the role of interneurons.
- Identify four specific functions of neuroglia.
- What is the relationship between the proportion of neuroglia to neurons and intelligence?
- l in the blanks:
________________ conduct nerve impulses, while _____________ support, nourish and create myelin sheath for these cells. In the central nervous system, the myelin sheath is produced by _________________, while in the peripheral nervous system, ________________ make the sheath. The tiny gap between an axon terminal and a neighboring dendrite is called a ________________. In some motor neurons, the axon terminal doesn’t carry an impulse to a neighboring neuron, but to a ________________.
8.4 NERVE IMPULSES
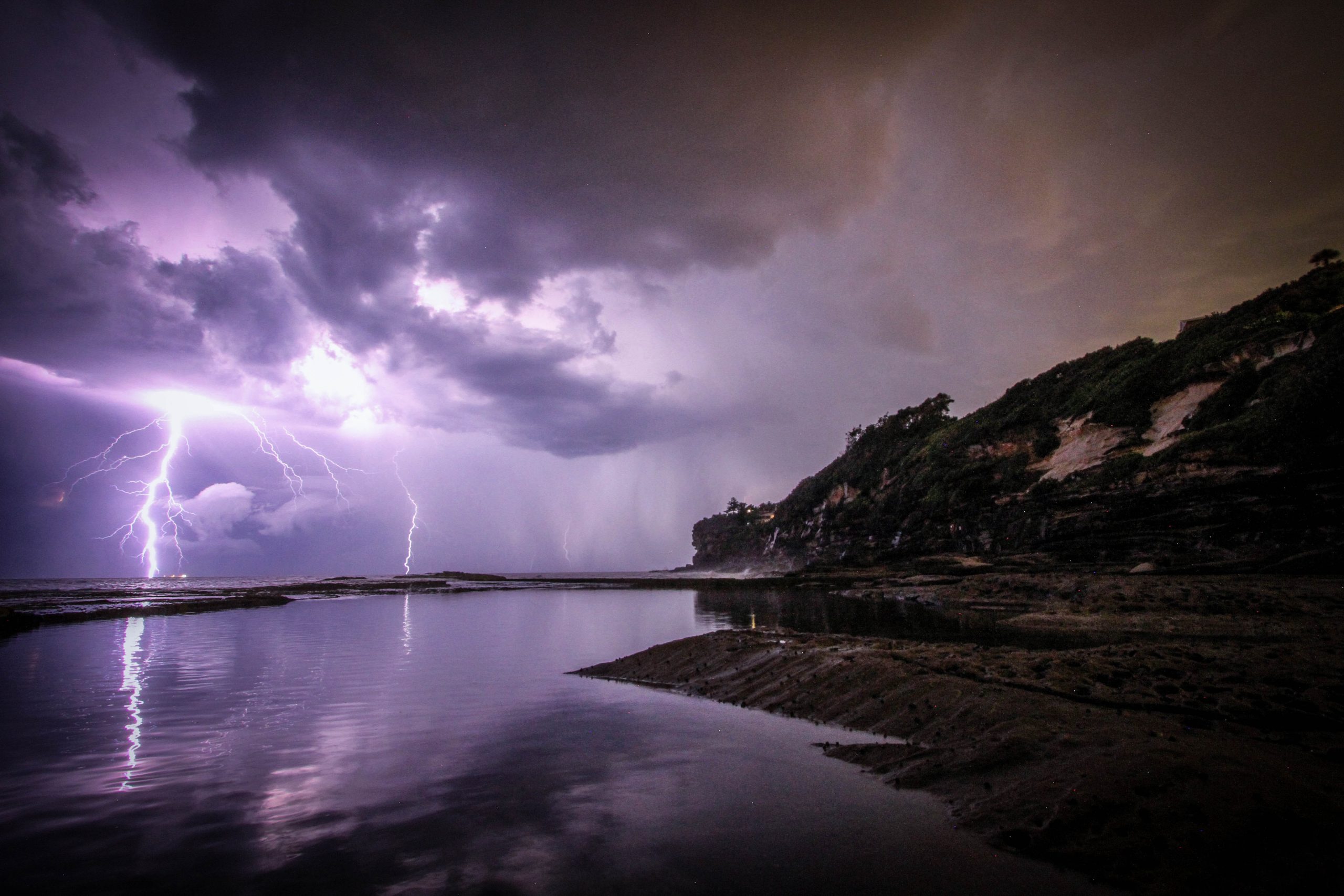
When Lightning Strikes
This amazing cloud-to-surface lightning occurred when a difference in electrical charge built up in a cloud relative to the ground. When the buildup of charge was great enough, a sudden discharge of electricity occurred. A nerve impulse is similar to a lightning strike. Both a nerve impulse and a lightning strike occur because of differences in electrical charge, and both result in an electric current.
GENERATING NERVE IMPULSES
A nerve impulse, like a lightning strike, is an electrical phenomenon. A nerve impulse occurs because of a difference in electrical charge across the plasma membrane of a neuron. How does this difference in electrical charge come about? The answer involves ions, which are electrically charged atoms or molecules.
RESTING POTENTIAL
When a neuron is not actively transmitting a nerve impulse, it is in a resting state, ready to transmit a nerve impulse. During the resting state, the sodium-potassium pump maintains a difference in charge across the cell membranes of the neuron. The sodium-potassium pump is a mechanism of active transport that moves sodium ions (Na+) out of cells and potassium ions (K+) into cells. The sodium-potassium pump moves both ions from areas of lower to higher concentration, using energy in ATP and carrier proteins in the cell membrane. The video below, “Sodium Potassium Pump” by Amoeba Sisters, describes in greater detail how the sodium-potassium pump works. Sodium is the principal ion in the fluid outside of cells, and potassium is the principal ion in the fluid inside of cells. These differences in concentration create an electrical gradient across the cell membrane, called resting potential. Tightly controlling membrane resting potential is critical for the transmission of nerve impulses.
Figure 8.12: The sodium-potassium pump maintains the resting potential of a neuron. There is more negative charge inside than outside the cell membrane. ATP is used to pump sodium out and potassium into the cell. There is more concentration of sodium outside the membrane and more concentration of potassium inside the cell due to the unequal movement of these ions by the pump
Watch the following video on the sodium-potassium pump as a review.
Sodium Potassium Pump, Amoeba Sisters, 2020.
ACTION POTENTIAL
A nerve impulse is a sudden reversal of the electrical gradient across the plasma membrane of a resting neuron. The reversal of charge is called an action potential. It begins when the neuron receives a chemical signal from another cell or some other type of stimulus. If the stimulus is strong enough to reach threshold, an action potential will take place is a cascade along the axon. There are three stages to an action potential:
1. Depolarization
As a result of the slight change in charge across the membrane voltage-gated sodium channels open and positively charged sodium ions (Na+) rush into the neuron. As these ions rush in, the inside of the cell membrane becomes positively charged as compared to the outside of the cell.
2. Repolarization
As a result of the positive charge created by depolarization, voltage-gated potassium channels open, and positively charged potassium ions (K+) rush out of the cell, reversing the polarity so that the inside of the cell is now negatively charged as compared to its surroundings.
3. Refractory/Recovery Period – Reestablishment of the Resting Potential
The sodium potassium pump works to restore resting potential by moving sodium ions out of the cell and potassium ions into the cell in a 3:2 ratio.
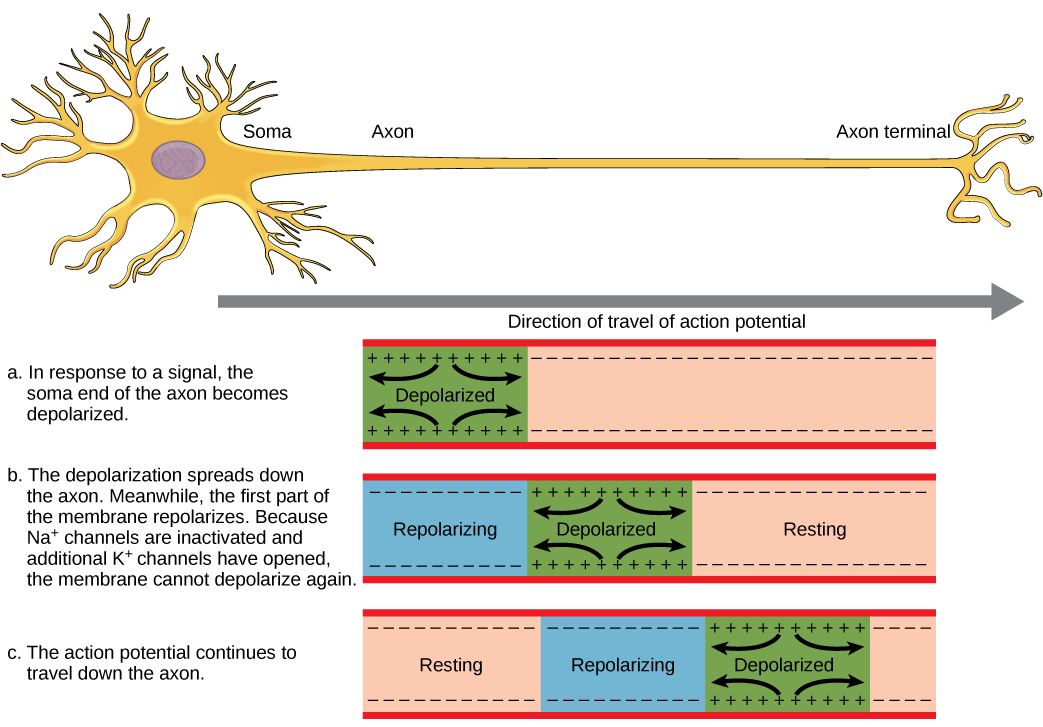
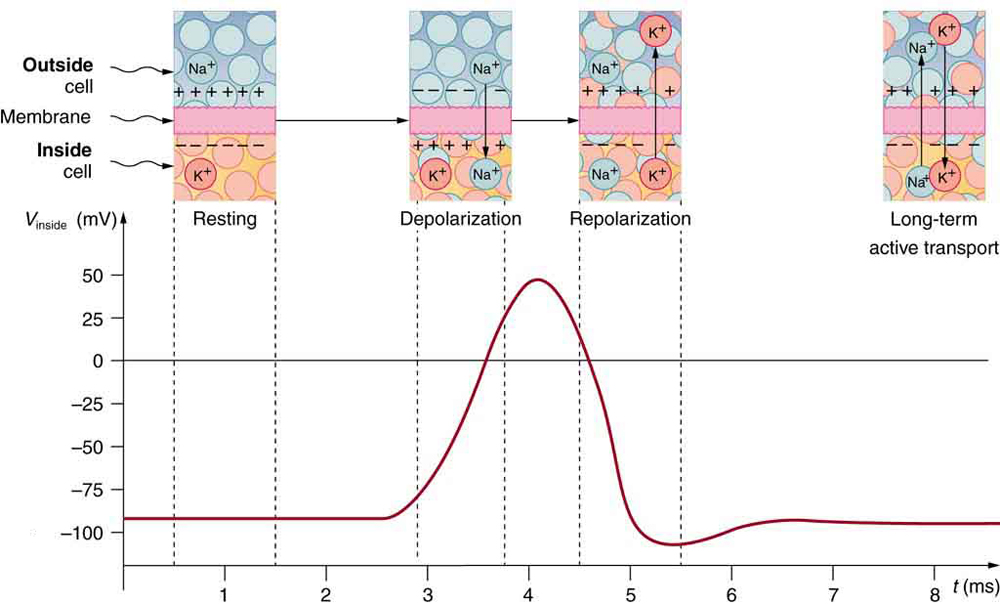
Figure 8.14: An action potential speeds along an axon in milliseconds. Sodium ions flow in and cause the action potential, and then potassium ions flow to
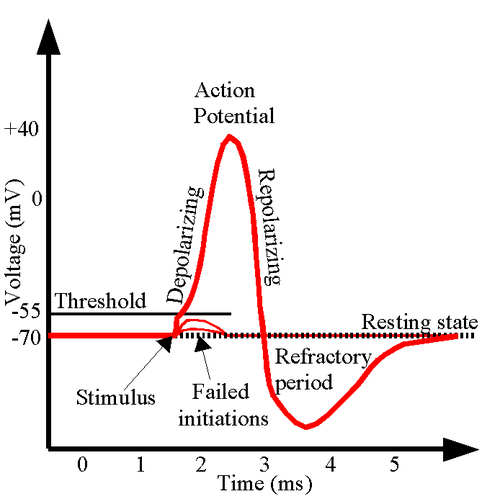
Figure 8.15 An action potential graph of membrane potential over time. A neuron must reach a certain threshold in order to begin the depolarization step of reaching the action potential. The figure also shows the change in potential during the repolarization and refractory periods of the axon.
The first channels to open are the sodium ion channels, which allow sodium ions to enter the cell. The resulting increase in positive charge inside the cell (up to about +40 mV) starts the action potential. This is called the depolarization of the membrane. Potassium ion channels then open, allowing potassium ions to flow out of the cell, which ends the action potential. The inside of the membrane becomes negative again. This is called repolarization of the membrane. Both of the ion channels then close, and the sodium-potassium pump restores the resting potential of -70 mV. The action potential will move down the axon toward the synapse like a wave would move along the surface of the water. Figure 8.14 shows the change in potential of the axon membrane during an action potential. The nerve goes through a brief refractory period before racing resting potential. During the refractory period, another action potential cannot be generated
In neurons with a myelin sheath on their axon, ions flow across the membrane only at the nodes between sections of myelin. As a result, the action potential appears to jump along the axon membrane from node to node, rather than spreading smoothly along the entire membrane. This increases the speed at which the action potential travels.
TRANSMITTING NERVE IMPULSES
The place where an axon terminal meets another cell is called a synapse. This is where the transmission of a nerve impulse to another cell occurs. The cell that sends the nerve impulse is called the presynaptic cell, and the cell that receives the nerve impulse is called the postsynaptic cell.
Some synapses are purely electrical and make direct electrical connections between neurons. Most synapses, however, are chemical synapses. Transmission of nerve impulses across chemical synapses is more complex.
CHEMICAL SYNAPSES
At a chemical synapse, both the presynaptic and postsynaptic areas of the cells are full of molecular machinery that is involved in the transmission of nerve impulses. As shown in Figure 8.16, the presynaptic area contains many tiny spherical vessels called synaptic vesicles that are packed with chemicals called neurotransmitters. When an action potential reaches the axon terminal of the presynaptic cell, it opens channels that allow calcium to enter the terminal. Calcium causes synaptic vesicles to fuse with the membrane, releasing their contents into the narrow space between the presynaptic and postsynaptic membranes. This area is called the synaptic cleft. The neurotransmitter molecules travel across the synaptic cleft and bind to receptors, which are proteins embedded in the membrane of the postsynaptic cell.
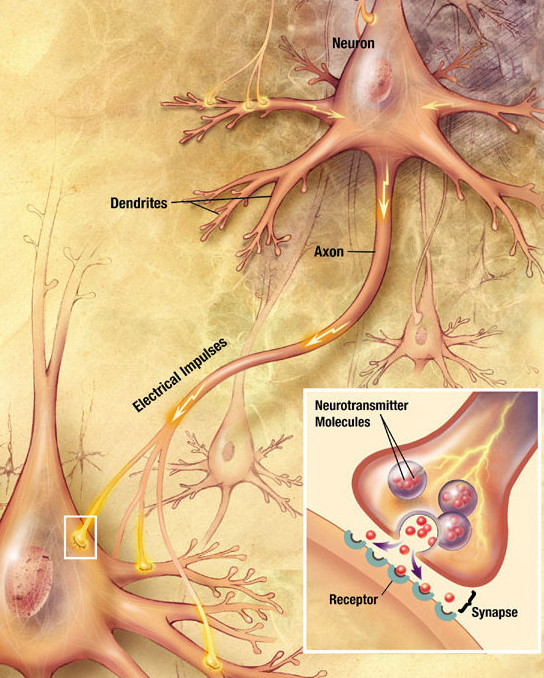
NEUROTRANSMITTERS AND RECEPTORS
There are more than a hundred known neurotransmitters, and more than one type of neurotransmitter may be released at a given synapse by a presynaptic cell. For example, it is common for a faster-acting neurotransmitter to be released, along with a slower-acting neurotransmitter. Many neurotransmitters also have multiple types of receptors to which they can bind. Receptors, in turn, can be divided into two general groups: chemically gated ion channels and second messenger systems.
- When a chemically gated ion channel is activated, it forms a passage that allows specific types of ions to flow across the cell membrane. Depending on the type of ion, the effect on the target cell may be excitatory or inhibitory.
- When a second messenger system is activated, it starts a cascade of molecular interactions inside the target cell. This may ultimately produce a wide variety of complex effects, such as increasing or decreasing the sensitivity of the cell to stimuli, or even altering gene transcription.
The effect of a neurotransmitter on a postsynaptic cell depends mainly on the type of receptors that it activates, making it possible for a particular neurotransmitter to have different effects on various target cells. A neurotransmitter might excite one set of target cells, inhibit others, and have complex modulatory effects on still others, depending on the type of receptors. However, some neurotransmitters have relatively consistent effects on other cells. Consider the two most widely used neurotransmitters, glutamate and GABA (gamma-aminobutyric acid). Glutamate receptors are either excitatory or modulatory in their effects, whereas GABA receptors are all inhibitory in their effects in adults.
Problems with neurotransmitters or their receptors can cause neurological disorders. The disease myasthenia gravis, for example, is caused by antibodies from the immune system blocking receptors for the neurotransmitter acetylcholine in postsynaptic muscle cells. This inhibits the effects of acetylcholine on muscle contractions, producing symptoms, such as muscle weakness and excessive fatigue during simple activities. Some mental illnesses (including depression) are caused, at least in part, by imbalances of certain neurotransmitters in the brain. One of the neurotransmitters involved in depression is thought to be serotonin, which normally helps regulate mood, among many other functions. Some antidepressant drugs are thought to help alleviate depression in many patients by normalizing the activity of serotonin in the brain.
Review
- Define nerve impulse.
- What is the resting potential of a neuron, and how is it maintained?
- Explain how and why an action potential occurs.
- Outline how a signal is transmitted from a presynaptic cell to a postsynaptic cell at a chemical synapse.
- What generally determines the effects of a neurotransmitter on a postsynaptic cell?
- Identify three general types of effects that neurotransmitters may have on postsynaptic cells.
- Explain how an electrical signal in a presynaptic neuron causes the transmission of a chemical signal at the synapse.
- The flow of which type of ion into a neuron results in an action potential? How do these ions get into the cell? What does this flow of ions do to the relative charge inside the neuron compared to the outside?
- Name three neurotransmitters.
- What are the three stages of an action potential? What ions are involved in each stage?
-
What is the function of the sodium-potassium pump on the cell membrane?
Action Potentials, Teacher’s Pet, 2018.
TED Ed| What is depression? – Helen M. Farrell, Parta Learning, 2017.
8.5 CENTRAL NERVOUS SYSTEM
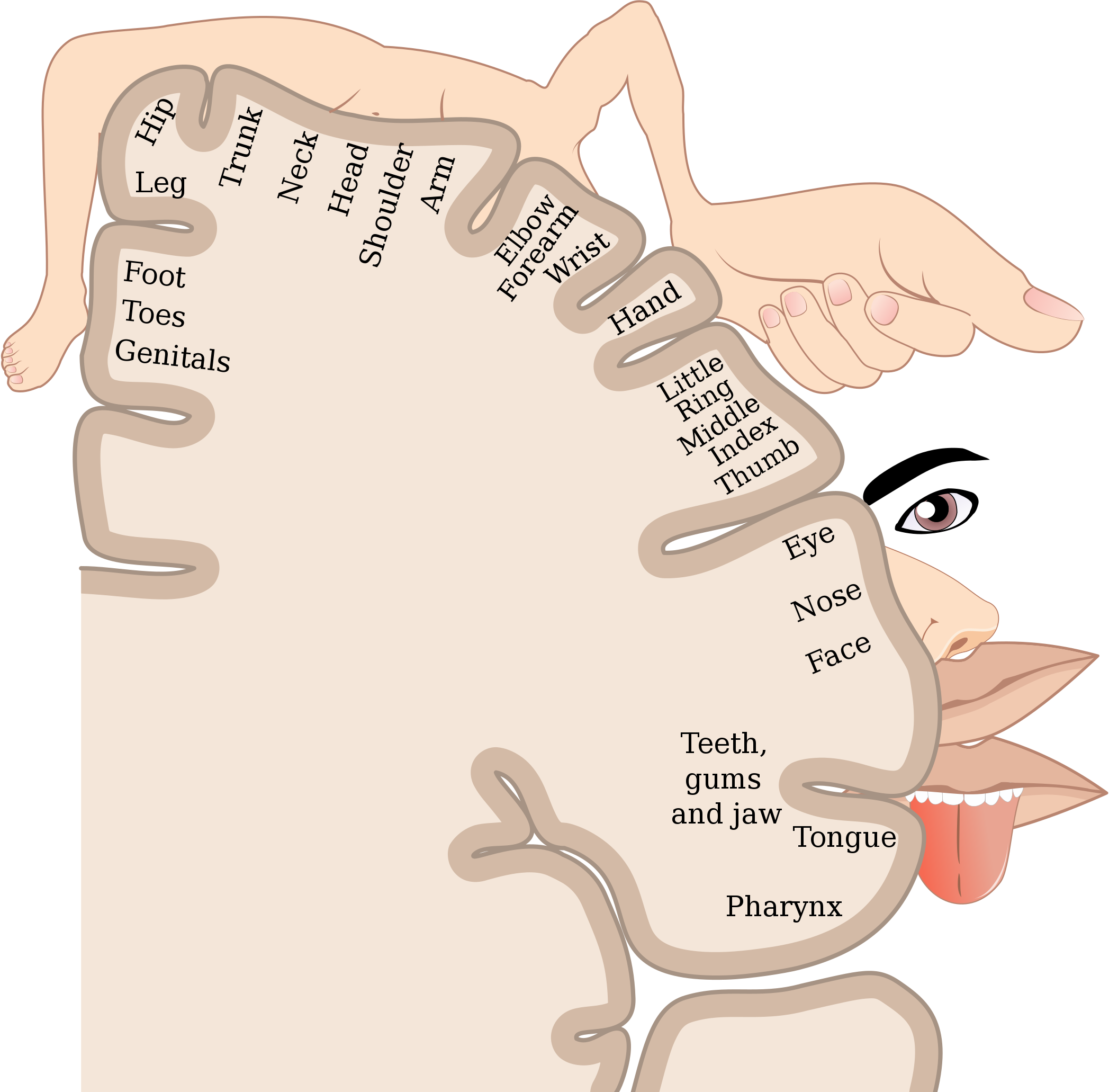
HOMUNCULUS
The very odd-looking drawing in Figure 17 is called a homunculus. The beige mass represents a cross-sectional wedge of the human brain, and the drawing shows some areas of the brain associated with different parts of the body. As you can see, larger areas of the brain in this region are associated with the hands, face, and tongue, as compared to the areas associated with the legs and feet. Given the importance of speech, manual dexterity, and face-to-face social interactions in human beings, it is not surprising that relatively large areas of the brain are needed to control these body parts. The brain is the most complex organ in the human body and part of the central nervous system.
WHAT IS THE CENTRAL NERVOUS SYSTEM?
The central nervous system (CNS) is the part of the nervous system that includes the brain and spinal cord. The drawing below (Figure 8.18shows the central nervous system as one of two main divisions of the total nervous system. The other main division is the peripheral nervous system (PNS). The CNS and PNS work together to control virtually all body functions.
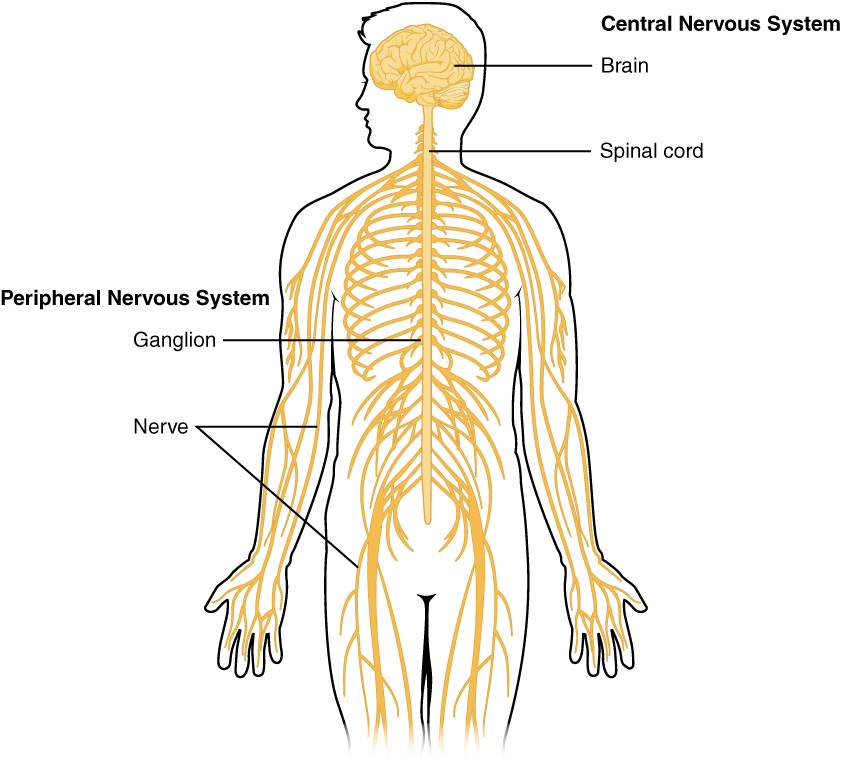
The delicate nervous tissues of the central nervous system are protected by major physical and chemical barriers. Physically, the brain and spinal cord are surrounded by tough meninges, a three-layer protective sheath that also contains cushioning cerebrospinal fluid. The bones of the skull and spinal vertebrae also contribute to physically protecting the brain and spinal cord. Chemically, the brain and spinal cord are isolated from the circulation — and most toxins or pathogens in the blood — by the blood-brain barrier. The blood-brain barrier is a highly selective membrane formed of endothelial cells that separates the circulating blood from extracellular fluid in the CNS. The barrier allows water, certain gases, glucose, and some other molecules needed by the brain and spinal cord to cross from the blood into the CNS, while keeping out potentially harmful substances. These physical and chemical barriers make the CNS less susceptible to injury than the PNS. However, damage to the CNS is likely to have more serious consequences.
THE BRAIN
The brain is the control center of not only the rest of the nervous system, but of the entire organism. The adult brain makes up only about 2% of the body’s weight, but it uses about 20% of the body’s total energy. The brain contains an estimated 100 billion neurons, and each neuron has thousands of synaptic connections to other neurons. The brain also has about the same number of neuroglia as neurons. No wonder the brain uses so much energy! In addition, the brain uses mostly glucose for energy. As a result, if the brain is deprived of glucose, it can lead to unconsciousness. The brain is able to store some glucose in the form of glycogen, but in much smaller amounts than are found in the liver and skeletal muscles.
The brain controls such mental processes as reasoning, imagination, memory, and language. It also interprets information from the senses and commands the body to respond appropriately. It controls basic physical processes (such as breathing and heartbeat), as well as voluntary activities (such as walking and writing). The brain has three major regions: the hindbrain, the midbrain and the forebrain. These parts are shown in Figure 8.19 and described below.
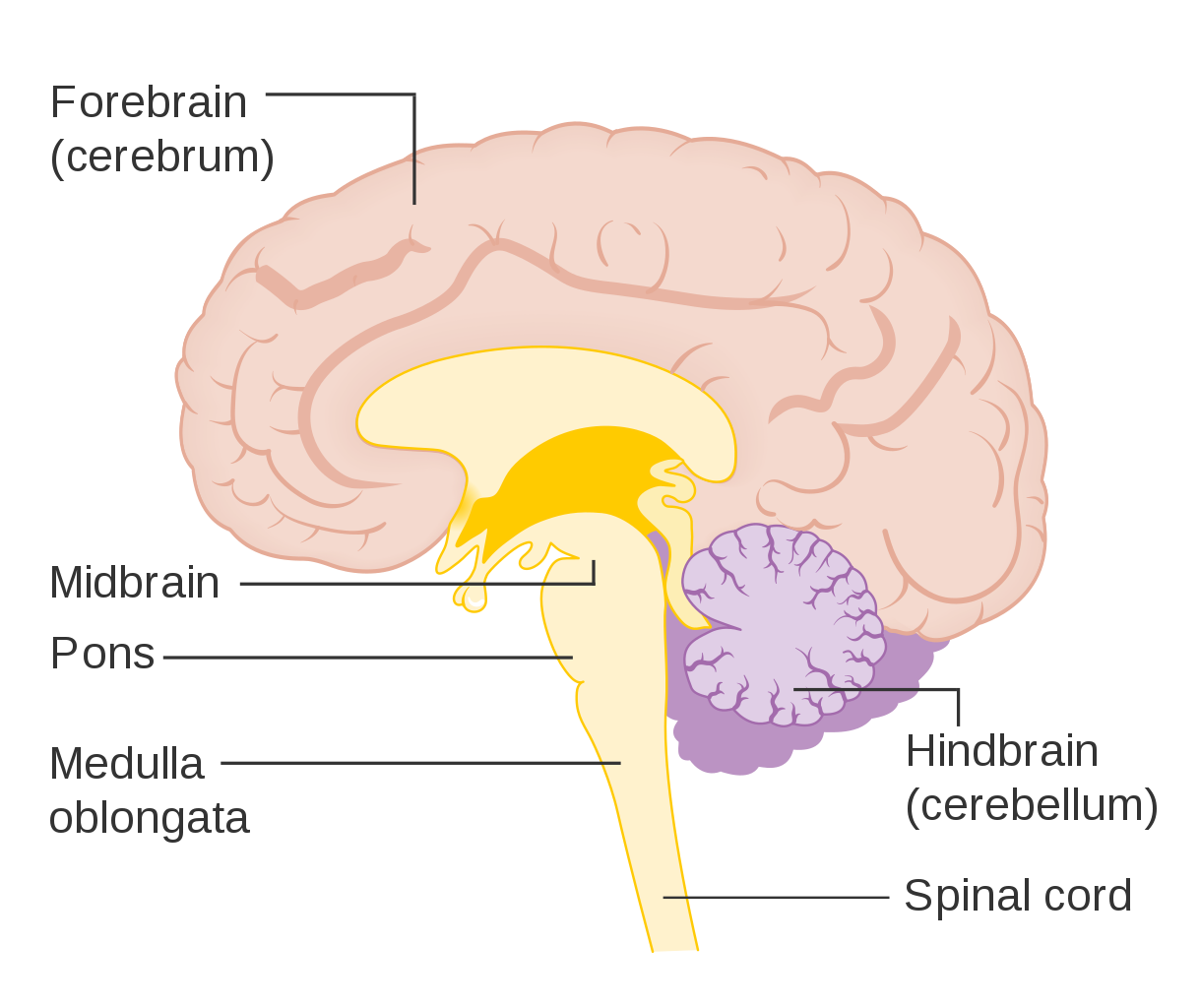
THE HINDBRAIN
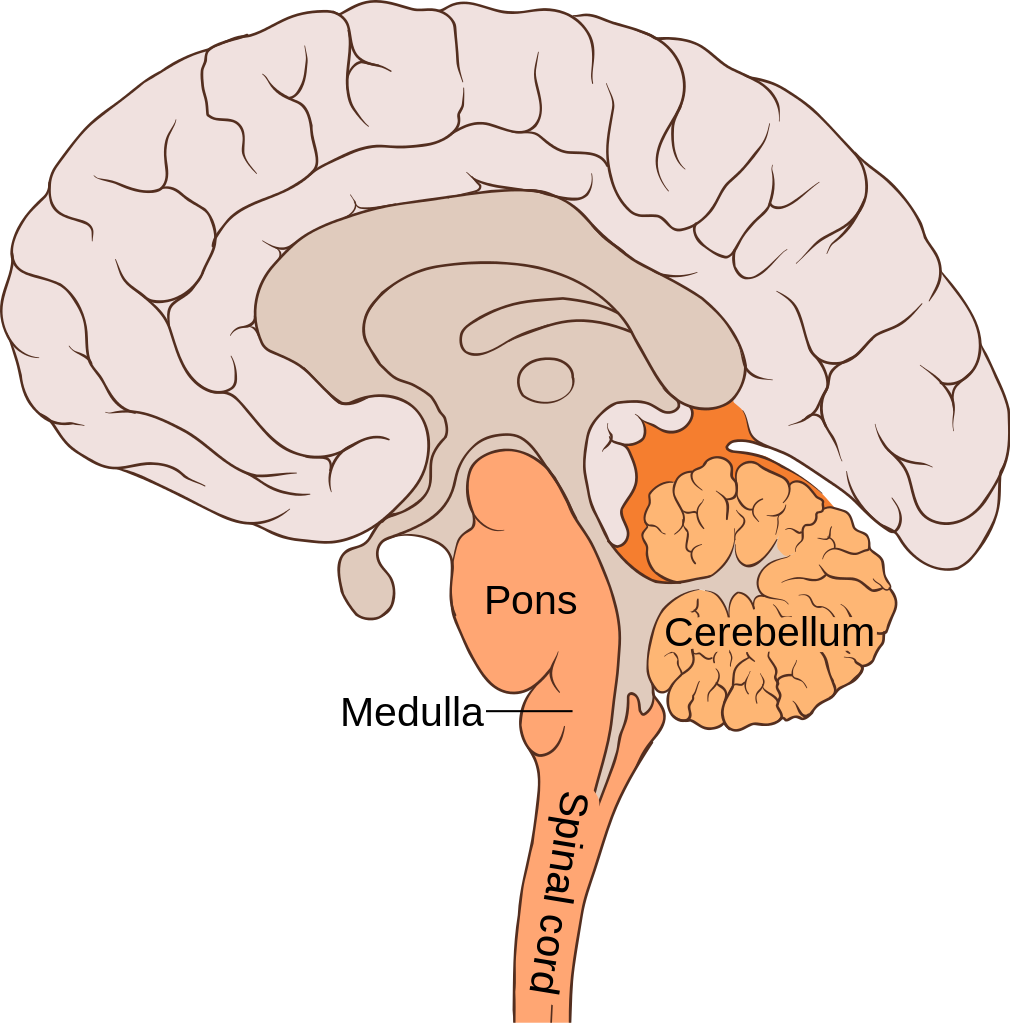
The hindbrain, which includes the cerebellum, medulla oblongata and the pons. The hindbrain is the lowest part of the brain. It resembles a stalk and platform on which the cerebrum is perched. The components of the hindbrain connect the rest of the brain with the spinal cord and passes nerve impulses between the brain and spinal cord.
Cerebellum
The cerebellum is located just below the cerebrum and at the back of the brain behind the brain stem. It coordinates your voluntary movements, balance, and posture. Information from your inner ear, joints and muscles, and eyes are all knitted together in the cerebellum so that you have awareness of where you are in 3-dimensional space. Patients who have suffered damage to their cerebellum may suffer from balance disorder. In addition, the cerebellum plays a major role in motor learning (like how to ride a bike or how to do a backflip on a trampoline) through trial and error. While traditionally, the cerebellum was thought to only be involved in motor functions, we now know that it also plays an important role in memory and learning.
Medulla Oblongata
The medulla oblongata makes up part of the brainstem and sits in front of and just below the cerebellum, at the very top of the spinal cord. It is responsible for control of heart rate, respiration rate and blood pressure, as well as reflexes such as vomiting, coughing, sneezing and swallowing.
Pons
The pons is located in front of the cerebellum and above the medulla oblongata. It has several functions, including receiving sensory information from the face, regulating rate and depth of breaths, as well as sleep cycles.
MIDBRAIN
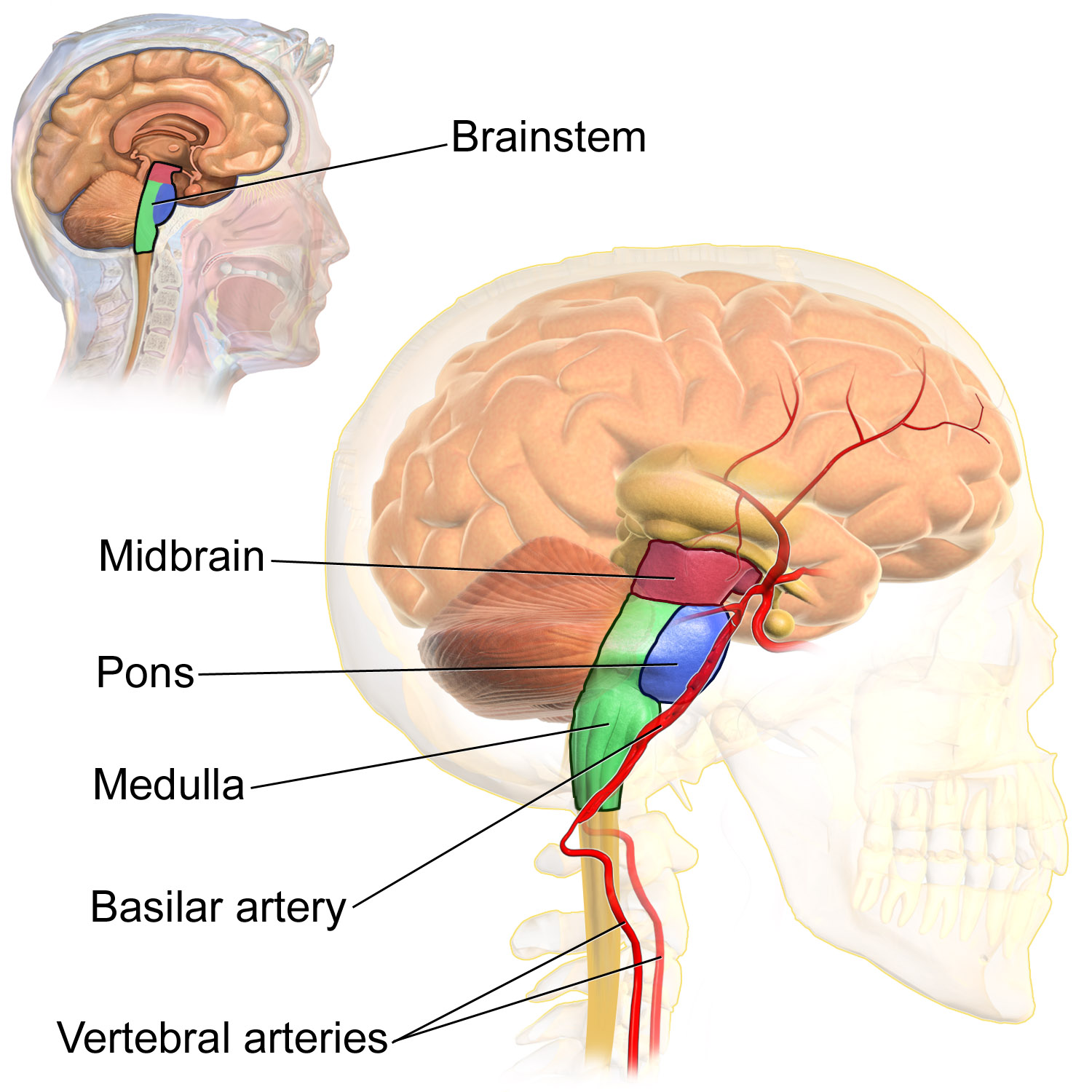
The midbrain is the topmost part of the brainstem and is the essential connection between and brain and spinal cord. The three main parts of the midbrain are the colliculi, the tegmentum, and the cerebral peduncles. These structures are all considered part of the brainstem, which consists of the medulla oblongata, the pons and the midbrain.
Reticular Activating System
The reticular activating system (RAS) is responsible for the sleep-wake cycle and wakefulness. It also regulates attention, ability to focus and arousal.
FOREBRAIN
The forebrain is the anterior (forwardmost) part of the brain and includes the cerebrum, the thalamus and hypothalamus, hippocampus, amygdala, and limbic system. This portion of the brain is responsible for processing incoming sensory information, performing complex cognitive activities (speech, abstract thought, etc.) and governing voluntary motor movements. The forebrain also controls body temperature, reproductive functions, eating, sleeping and the display of emotions.
Cerebrum
The cerebrum is the largest part of the brain. It controls conscious, intellectual functions. Among other things, it controls reasoning, language, memory, sight, touch, and hearing. When you read a book, play a video game, or recognize a classmate, you are using your cerebrum.
Hemispheres and Lateralization of the Cerebrum
The cerebrum is divided from front to back into two halves called the left and right hemispheres. The two hemispheres are connected by a thick bundle of axons, known as the corpus callosum, which lies deep within the brain. The corpus callosum is the main avenue of communication between the two hemispheres. It connects each point in the cerebrum to the mirror-image point in the opposite hemisphere.
The right and left hemispheres of the cerebrum are similar in shape, and most areas of cerebrum are found in both hemispheres. Some areas, however, show laterization, or a concentration in one hemisphere or the other. In most people, for example, language functions are more concentrated in the left hemisphere, whereas abstract reasoning and visual-spatial abilities are more concentrated in the right hemisphere.
For reasons that are not yet clear, each hemisphere of the brain interacts primarily with the opposite side of the body. The left side of the brain receives messages from and sends commands to the right side of the body, and the right side of the brain receives messages from and sends commands to the left side of the body. Sensory nerves from the spinal cord to the brain and motor nerves from the brain to the spinal cord both cross the midline of the body at the level of the brain stem.
Lobes of the Cerebrum
Each hemisphere of the cerebrum is further divided into the four lobes shown in Figure 8.22 and described below.
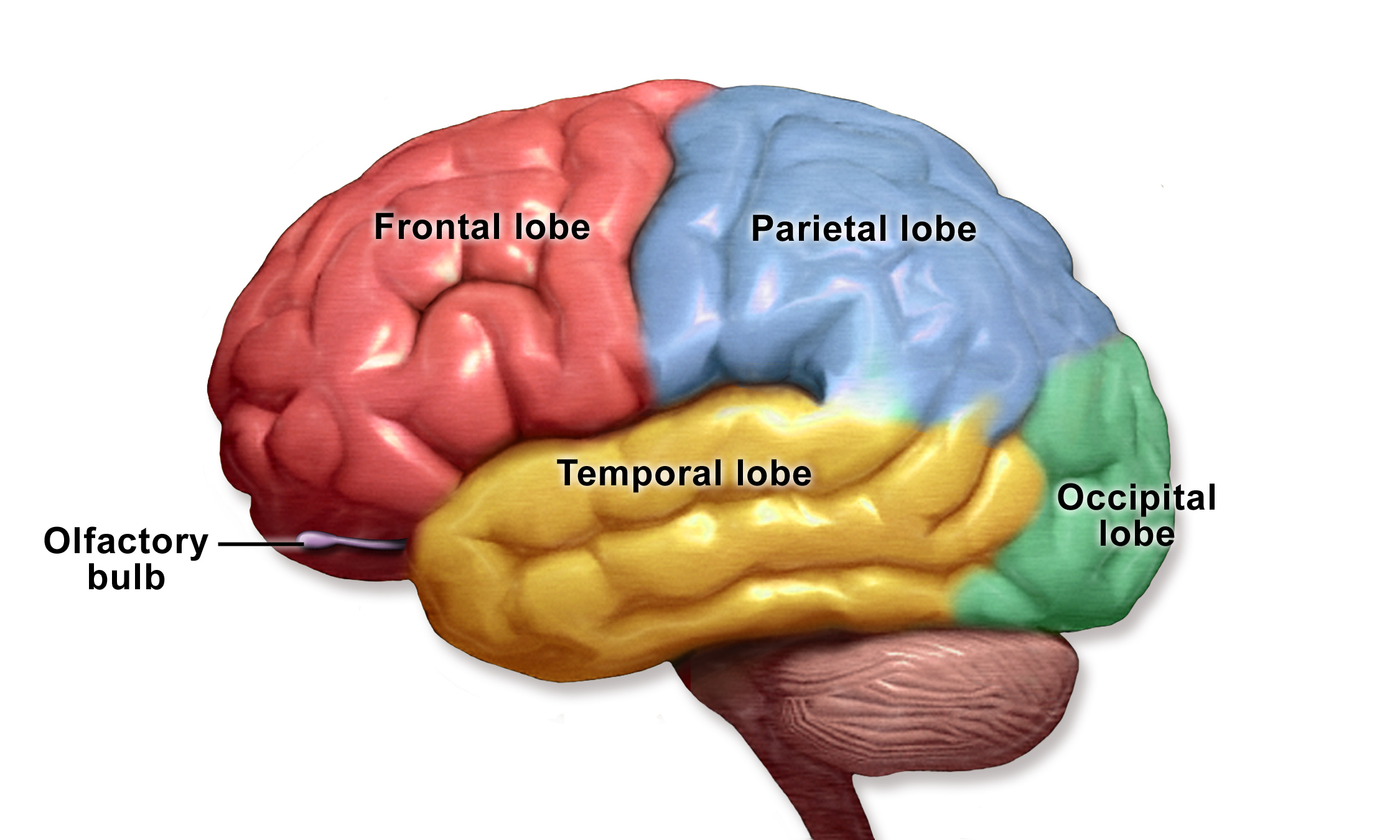
Each hemisphere of the cerebrum consists of four parts, called lobes. Each lobe is associated with particular brain functions. Just one function of each lobe is listed here.
- The frontal lobes are located at the front of the brain behind the forehead. The frontal lobes are associated with executive functions, such as attention, self-control, planning, problem solving, reasoning, abstract thought, language, and personality.
- The parietal lobes are located behind the frontal lobes at the top of the head. The parietal lobes are involved in sensation — including temperature, touch, and taste. Reading and arithmetic are also functions of the parietal lobes.
- The temporal lobes are located at the sides of the head below the frontal and parietal lobes. The temporal lobes enable hearing, the formation and retrieval of memories, and the integration of memories and sensations.
- The occipital lobes are located at the back of the head below the parietal lobes. The occipital lobes are the smallest of the four pairs of lobes. They are dedicated almost solely to vision.
Cerebral Cortex
Most of the information processing in the brain actually takes place in the cerebral cortex, a rind of gray matter and other tissues just a few millimeters thick that makes up the outer surface of the cerebrum in both hemispheres of the brain. The cerebral cortex has many folds in it, greatly increasing the amount of surface area of the brain that can fit within the skull. Because of all the folds in the human cerebral cortex, it has a surface area of about 2,500 cm2 (2.5 ft2). The size and importance of the cerebral cortex is far greater in the human brain than the brains of any other vertebrates, including nonhuman primates.
The Limbic System
The limbic system consists of the hypothalamus, thalamus, the hippocampus and amygdala. The structures and interacting areas of the limbic system are involved in motivation, emotion, learning, and memory.
Thalamus and Hypothalamus
Several structures are located deep within the brain and are important for communication between the brain and spinal cord (or the rest of the body). These structures include the hypothalamus and thalamus. The diagram below (Figure 8.5.7) shows where these structures are located in the brain. Like the two halves of the cerebrum, the hypothalamus and thalamus exist in two halves, one in each hemisphere.
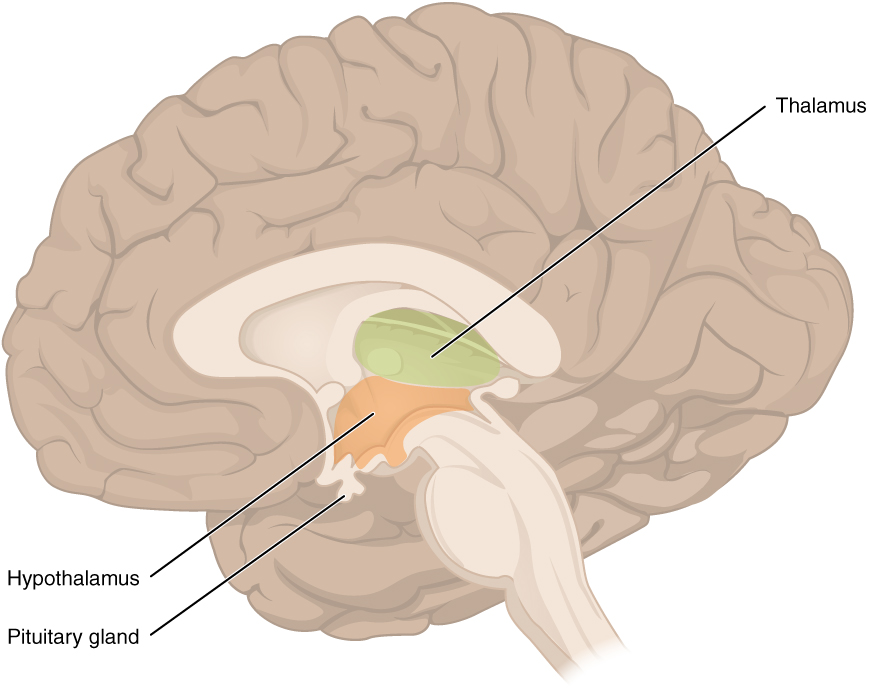
The hypothalamus is located just above the brain stem and is about the size of an almond. The hypothalamus is responsible for certain metabolic processes and other activities of the autonomic nervous system, including body temperature, heart rate, hunger, thirst, fatigue, sleep, wakefulness, and circadian (24-hour) rhythms. The hypothalamus is also an important emotional center of the brain. The hypothalamus can regulate so many body functions because it responds to many different internal and external signals, including messages from the brain, light, steroid hormones, stress, and invading pathogens, among others.
One way the hypothalamus influences body functions is by synthesizing hormones that directly influence body processes. It synthesizes the hormone oxytocin, which stimulates uterine contractions during childbirth and the letdown of milk during lactation. It also synthesizes antidiuretic hormone, which stimulates the kidneys to reabsorb more water and excrete more concentrated urine. These two hormones are sent from the hypothalamus via a stalk-like structure called the infundibulum (see Figure 8.5.7) directly to the posterior (back) portion of the pituitary gland, which secretes them into the blood.
The main way the hypothalamus influences body functions is by controlling the pituitary gland, known as the master gland of the endocrine system. The hypothalamus synthesizes neurohormones called releasing factors that travel through the infundibulum directly to the anterior (front) part of the pituitary gland. The releasing factors generally either stimulate or inhibit the secretion of anterior pituitary hormones, most of which control other glands of the endocrine system.
The thalamus, which is located near the hypothalamus (see Figure 8.23), is a major hub for information traveling back and forth between the spinal cord and cerebrum. It relays sensory signals to the cerebral cortex and motor signals to the spinal cord. It is also involved in the regulation of consciousness, sleep, and alertness.
Hippocampus and Amygdala
The hippocampus a complex structure embedded deep in the temporal lobe. It plays a major role in learning and memory and contributes to regulation of motivation and emotion. The amygdala is the part of the brain responsible for formation and storage of memories associated with emotional events.
Watch “The Limbic System” by Soton Brain Hub to learn about the location and functions of the limbic system.
The Limbic System, Soton Brain Hub, 2016.
SPINAL CORD
The spinal cord is a long, thin, tubular bundle of nervous tissues that extends from the brain stem and continues down the center of the back to the pelvis. It is highlighted in yellow in Figure 8.24. The spinal cord is enclosed within, but is shorter than, the vertebral column.
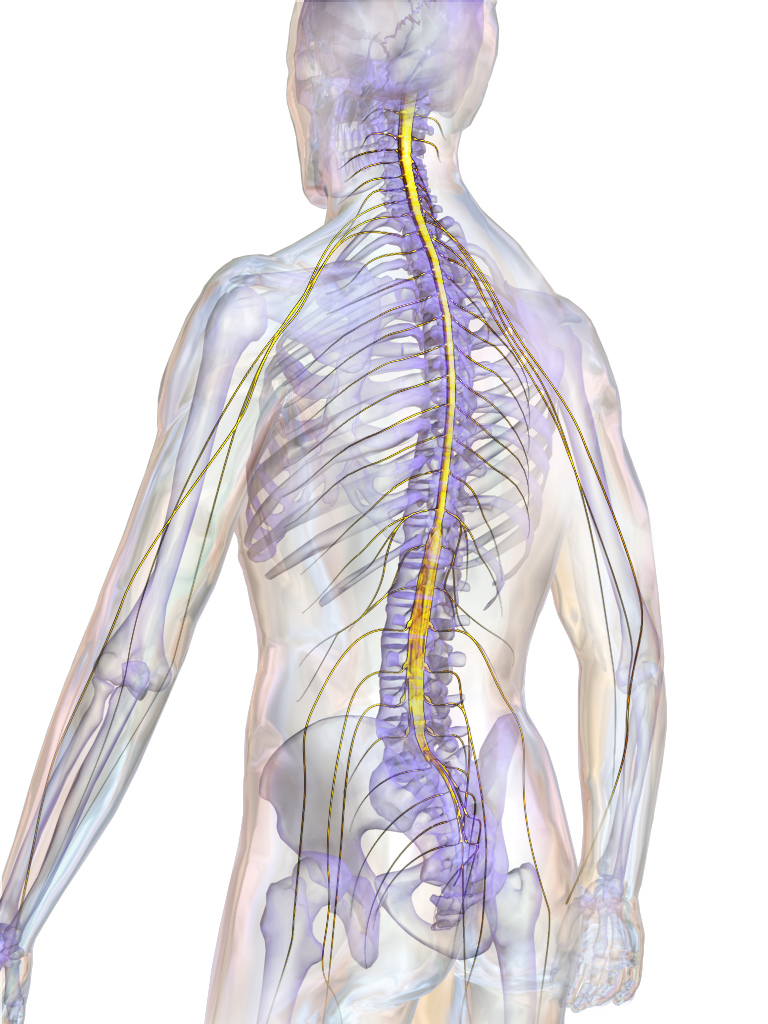
STRUCTURE OF THE SPINAL CORD
The center of the spinal cord consists of gray matter, which is made up mainly of cell bodies of neurons, including interneurons and motor neurons. The gray matter is surrounded by white matter that consists mainly of myelinated axons of motor and sensory neurons. Spinal nerves, which connect the spinal cord to the PNS, exit from the spinal cord between vertebrae (see Figure 8.25).
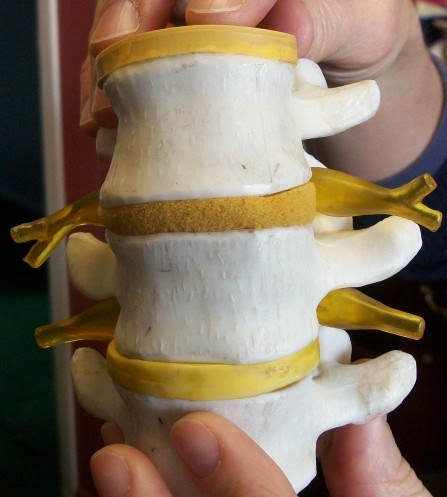
FUNCTIONS OF THE SPINAL CORD
The spinal cord serves as an information superhighway. It passes messages from the body to the brain and from the brain to the body. Sensory (afferent) nerves carry nerve impulses to the brain from sensory receptor cells everywhere in and on the body. Motor (efferent) nerves carry nerve impulses away from the brain to glands, organs, or muscles throughout the body.
The spinal cord also independently controls certain rapid responses called reflexes without any input from the brain. You can see how this may happen in Figure 8.26. A sensory receptor responds to a sensation and sends a nerve impulse along a sensory nerve to the spinal cord. In the spinal cord, the message passes to an interneuron and from the interneuron to a motor nerve, which carries the impulse to a muscle. The muscle contracts in response. These neuron connections form a reflex arc, which requires no input from the brain. No doubt you have experienced such reflex actions yourself. For example, you may have reached out to touch a pot on the stove, not realizing that it was very hot. Virtually at the same moment that you feel the burning heat, you jerk your arm back and remove your hand from the pot.
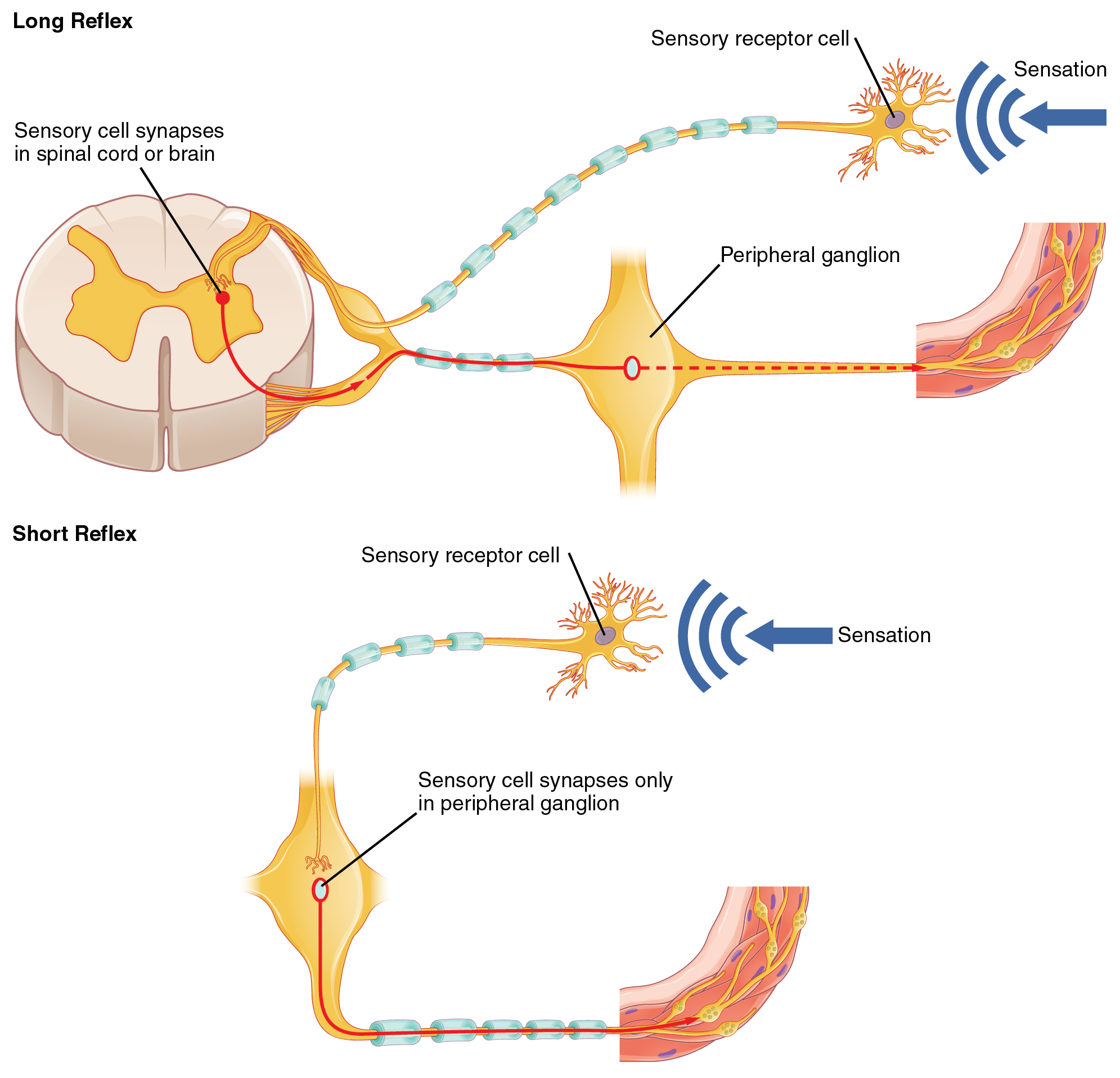
INJURIES TO THE SPINAL CORD
Physical damage to the spinal cord may result in paralysis, which is loss of sensation and movement in part of the body. Paralysis generally affects all the areas of the body below the level of the injury, because nerve impulses are interrupted and can no longer travel back and forth between the brain and body beyond that point. If an injury to the spinal cord produces nothing more than swelling, the symptoms may be transient. However, if nerve fibers (axons) in the spinal cord are badly damaged, the loss of function may be permanent. Experimental studies have shown that spinal nerve fibers attempt to regrow, but tissue destruction usually produces scar tissue that cannot be penetrated by the regrowing nerves, as well other factors that inhibit nerve fiber regrowth in the central nervous system.
Review
- What is the central nervous system?
- How is the central nervous system protected?
- What is the overall function of the brain?
- Identify the three main parts of the brain and one function of each part.
- Describe the hemispheres of the brain.
- Explain and give examples of lateralization of the brain.
- Identify one function of each of the four lobes of the cerebrum.
- Summarize the function of each of the parts of the brain.
- Summarize the structure and function of the cerebral cortex. Explain how the hypothalamus controls the endocrine system.
- Describe the spinal cord.
- What is the main function of the spinal cord?
- Explain how reflex actions occur.
- Why do severe spinal cord injuries usually cause paralysis?
- What do you think are some possible consequences of severe damage to the brain stem? How might this compare to the consequences of severe damage to the frontal lobe? Explain your answer.
- Information travels very quickly in the nervous system, but generally, the longer the path between areas, the longer it takes. Based on this, explain why you think reflexes often occur at the spinal cord level, and do not require input from the brain.
8.6 PERIPHERAL NERVOUS SYSTEM
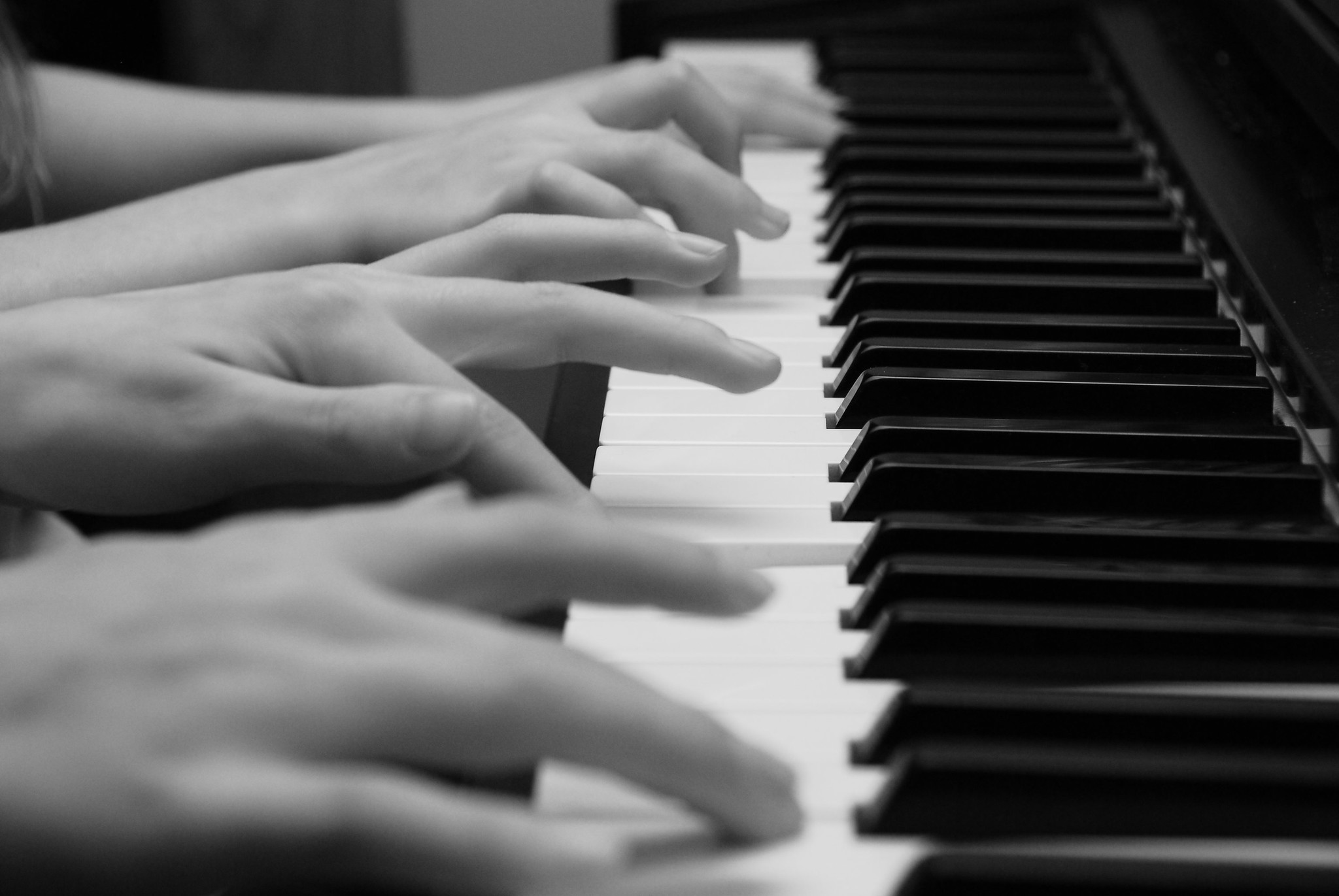
ONE PIANO, FOUR HANDS
Did you ever see two people play the same piano? How do they coordinate all the movements of their own fingers — let alone synchronize them with those of their partner? The peripheral nervous system plays an important part in this challenge.
WHAT IS THE PERIPHERAL NERVOUS SYSTEM?
The peripheral nervous system (PNS) consists of all the nervous tissue that lies outside of the central nervous system (CNS). The main function of the PNS is to connect the CNS to the rest of the organism. It serves as a communication relay, going back and forth between the CNS and muscles, organs, and glands throughout the body.
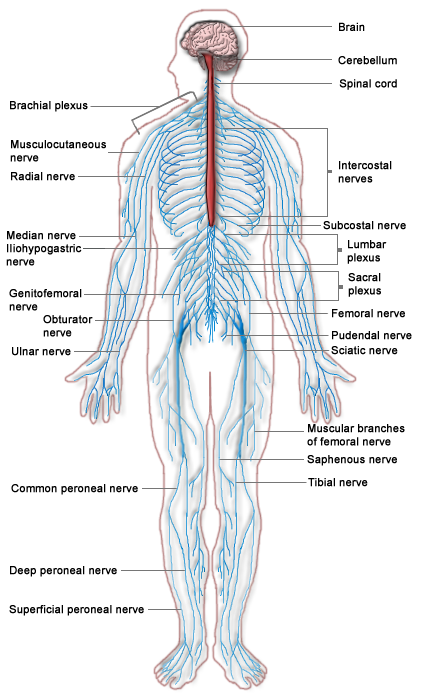
The PNS is mostly made up of cable-like bundles of axons called nerves, as well as clusters of neuronal cell bodies called ganglia (singular, ganglion). Nerves are generally classified as sensory, motor, or mixed nerves based on the direction in which they carry nerve impulses.
- Sensory nerves transmit information from sensory receptors in the body to the CNS. Sensory nerves are also called afferent nerves. You can see an example in the figure below.
- Motor nerves transmit information from the CNS to muscles, organs, and glands. Motor nerves are also called efferent nerves. You can see one in the figure below.
- Mixed nerves contain both sensory and motor neurons, so they can transmit information in both directions. They have both afferent and efferent functions.
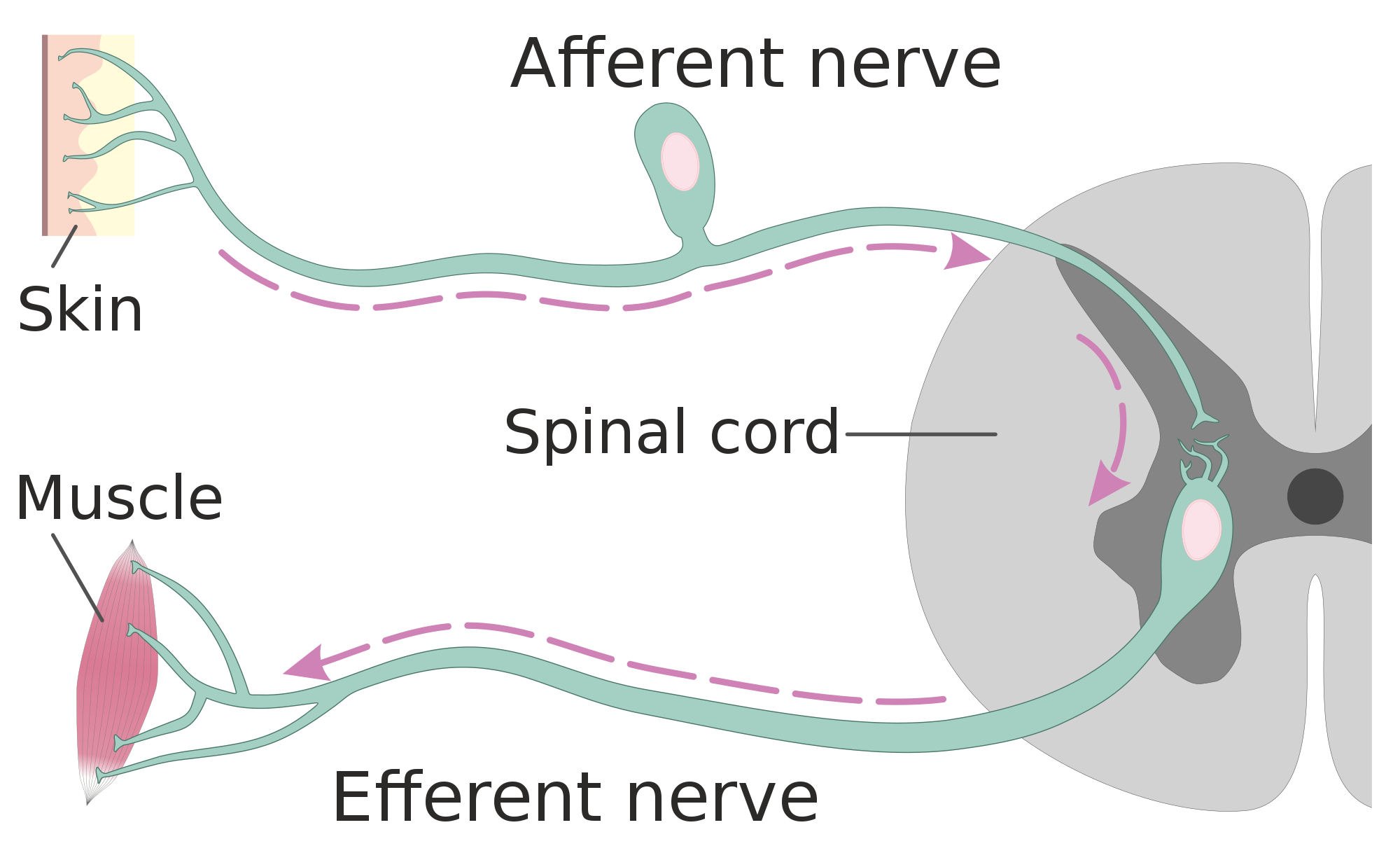
DIVISIONS OF THE PERIPHERAL NERVOUS SYSTEM
The PNS is divided into two major systems, called the autonomic nervous system and the somatic nervous system. In the diagram below, the autonomic system is shown on the left, and the somatic system on the right. Both systems of the PNS interact with the CNS and include sensory and motor neurons, but they use different circuits of nerves and ganglia.
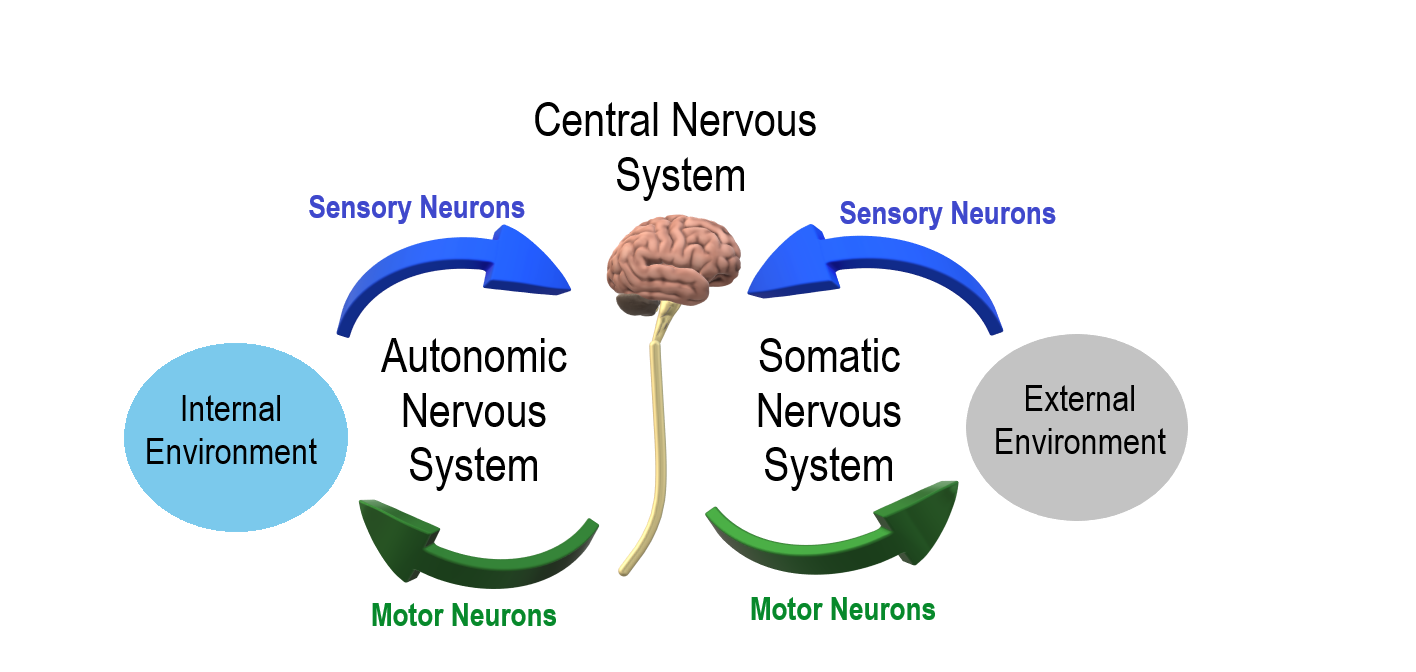
SOMATIC NERVOUS SYSTEM
The somatic nervous system primarily senses the external environment and controls voluntary activities about which decisions and commands come from the cerebral cortex of the brain. When you feel too warm, for example, you decide to turn on the air conditioner. As you walk across the room to the thermostat, you are using your somatic nervous system. In general, the somatic nervous system is responsible for all of your conscious perceptions of the outside world, as well as all of the voluntary motor activities you perform in response. Whether it’s playing a piano, driving a car, or playing basketball, you can thank your somatic nervous system for making it possible.
Somatic sensory and motor information is transmitted through 12 pairs of cranial nerves and 31 pairs of spinal nerves. Cranial nerves are in the head and neck and connect directly to the brain. Sensory components of cranial nerves transmit information about smells, tastes, light, sounds, and body position. Motor components of cranial nerves control skeletal muscles of the face, tongue, eyeballs, throat, head, and shoulders. Motor components of cranial nerves also control the salivary glands and swallowing. Four of the 12 cranial nerves participate in both sensory and motor functions as mixed nerves, having both sensory and motor neurons.
Spinal nerves emanate from the spinal column between vertebrae. All of the spinal nerves are mixed nerves, containing both sensory and motor neurons. The areas of skin innervated by the 31 pairs of spinal nerves are shown in the figure below. These include sensory nerves in the skin that sense pressure, temperature, vibrations, and pain. Other sensory nerves are in the muscles, and they sense stretching and tension. Spinal nerves also include motor nerves that stimulate skeletal muscles to contract, allowing for voluntary body movements.
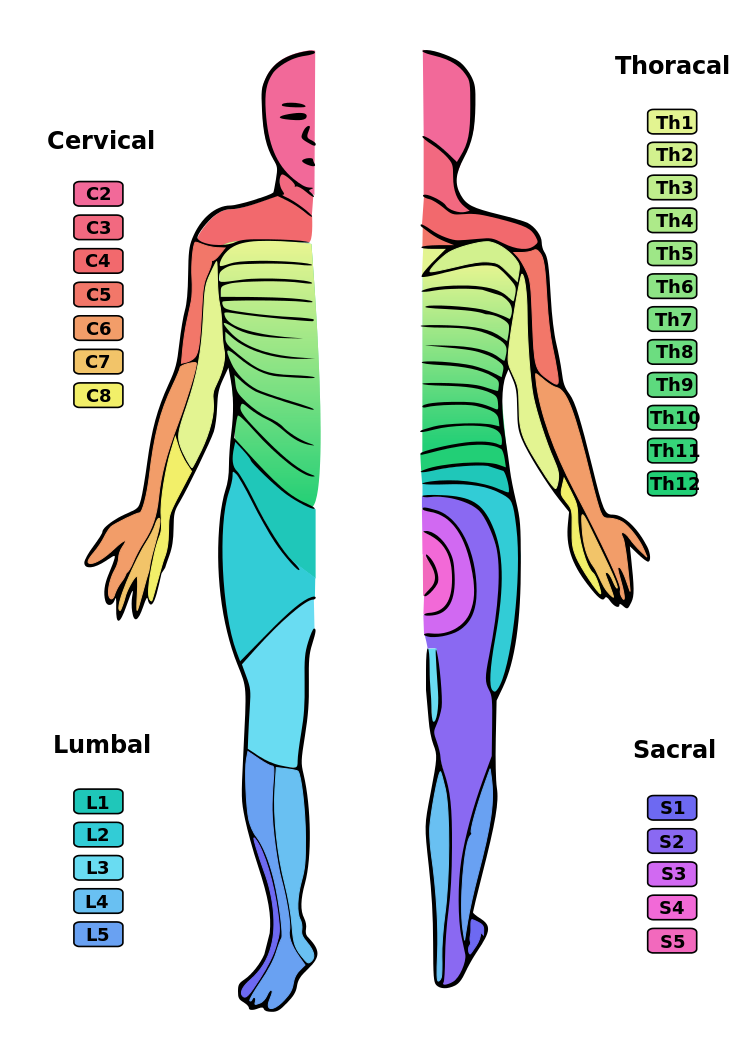
AUTONOMIC NERVOUS SYSTEM
The autonomic nervous system primarily senses the internal environment and controls involuntary activities. It is responsible for monitoring conditions in the internal environment and bringing about appropriate changes in them. In general, the autonomic nervous system is responsible for all the activities that go on inside your body without your conscious awareness or voluntary participation.
Structurally, the autonomic nervous system consists of sensory and motor nerves that run between the CNS (especially the hypothalamus in the brain), internal organs (such as the heart, lungs, and digestive organs), and glands (such as the pancreas and sweat glands). Sensory neurons in the autonomic system detect internal body conditions and send messages to the brain. Motor nerves in the autonomic system affect appropriate responses by controlling contractions of smooth or cardiac muscle, or glandular tissue. For example, when sensory nerves of the autonomic system detect a rise in body temperature, motor nerves signal smooth muscles in blood vessels near the body surface to undergo vasodilation, and the sweat glands in the skin to secrete more sweat to cool the body.
The autonomic nervous system, in turn, has three subdivisions: the sympathetic, parasympathetic and enteric divisions. The first two subdivisions of the autonomic system are summarized in the figure below. Both affect the same organs and glands, but they generally do so in opposite ways.
- The sympathetic division controls the fight-or-flight response. Changes occur in organs and glands throughout the body that prepare the body to fight or flee in response to a perceived danger. For example, the heart rate speeds up, air passages in the lungs become wider, more blood flows to the skeletal muscles, and the digestive system temporarily shuts down.
- The parasympathetic division returns the body to normal after the fight-or-flight response has occurred. For example, it slows down the heart rate, narrows air passages in the lungs, reduces blood flow to the skeletal muscles, and stimulates the digestive system to start working again. The parasympathetic division also maintains internal homeostasis of the body at other times.
- The enteric division is made up of nerve fibers that supply the organs of the digestive system. This division allows for the local control of many digestive functions.
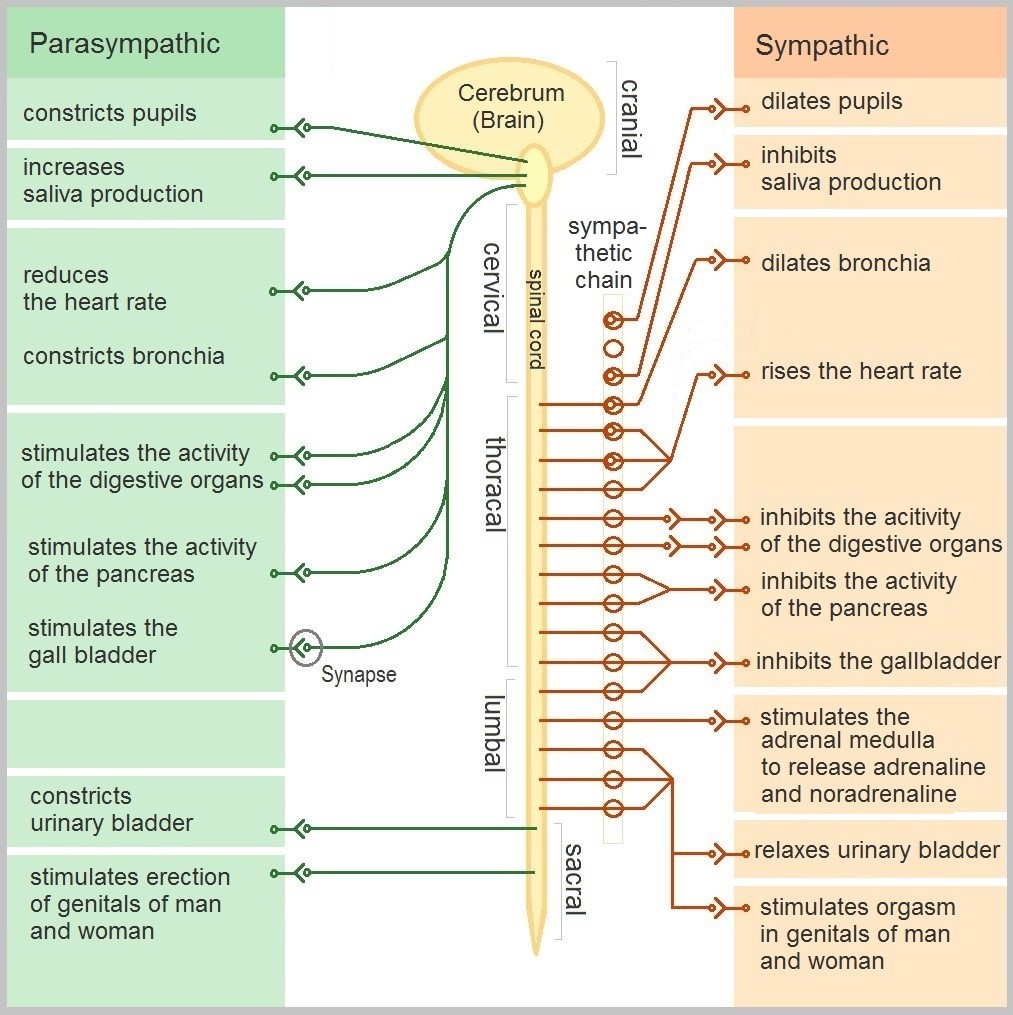
Disorders of the Peripheral Nervous System
Unlike the CNS — which is protected by bones, meninges and cerebrospinal fluid — the PNS has no such protections. The PNS also has no blood-brain barrier to protect it from toxins and pathogens in the blood. Therefore, the PNS is more subject to injury and disease than is the CNS. Causes of nerve injury include diabetes, infectious diseases (such as shingles), and poisoning by toxins (such as heavy metals). PNS disorders often have symptoms like loss of feeling, tingling, burning sensations, or muscle weakness. If a traumatic injury results in a nerve being transected (cut all the way through), it may regenerate, but this is a very slow process and may take many months.
Two other diseases of the PNS are Guillain-Barre syndrome and Charcot-Marie-Tooth disease.
- Guillain-Barre syndrome is a rare disease in which the immune system attacks nerves of the PNS, leading to muscle weakness and even paralysis. The exact cause of Guillain-Barre syndrome is unknown, but it often occurs after a viral or bacterial infection. There is no known cure for the syndrome, but most people eventually make a full recovery. Recovery can be slow, however, lasting anywhere from several weeks to several years.
- Charcot-Marie-Tooth disease is a hereditary disorder of the nerves, and one of the most common inherited neurological disorders. It affects predominantly the nerves in the feet and legs, and often in the hands and arms, as well. The disease is characterized by loss of muscle tissue and sense of touch. It is presently incurable.
FEATURE: MY HUMAN BODY
The autonomic nervous system is considered to be involuntary because it doesn’t require conscious input. However, it is possible to exert some voluntary control over it. People who practice yoga or other so-called mind-body techniques, for example, can reduce their heart rate and certain other autonomic functions. Slowing down these otherwise involuntary responses is a good way to relieve stress and reduce the wear-and-tear that stress can place on the body. Such techniques may also be useful for controlling post-traumatic stress disorder and chronic pain. Three types of integrative practices for these purposes are breathing exercises, body-based tension modulation exercises, and mindfulness techniques.
Breathing exercises can help control the rapid, shallow breathing that often occurs when you are anxious or under stress. These exercises can be learned quickly, and they provide immediate feelings of relief. Specific breathing exercises include paced breath, diaphragmatic breathing, and Breathe2Relax or Chill Zone on MindShift™ CBT, which are downloadable breathing practice mobile applications, or “Apps”.
Body-based tension modulation exercises include yoga postures (also known as “asanas”) and tension manipulation exercises. The latter include the Trauma/Tension Release Exercise (TRE) and the Trauma Resiliency Model (TRM).
Mindfulness techniques have been shown to reduce symptoms of depression, as well as those of anxiety and stress. They have also been shown to be useful for pain management and performance enhancement. Specific mindfulness programs include Mindfulness Based Stress Reduction (MBSR) and Mindfulness Mind-Fitness Training (MMFT). You can learn more about MBSR by watching the video below.
Mindfulness-Based Stress Reduction (UMass Medical School, Center for Mindfulness), Palouse Mindfulness, 2017.
Review
- Describe the general structure of the peripheral nervous system. State its primary function.
- What are ganglia?
- Identify three types of nerves based on the direction in which they carry nerve impulses.
- Outline all of the divisions of the peripheral nervous system.
- Compare and contrast the somatic and autonomic nervous systems.
- When and how does the sympathetic division of the autonomic nervous system affect the body?
- What is the function of the parasympathetic division of the autonomic nervous system? Specifically, how does it affect the body?
- Name and describe two peripheral nervous system disorders.
- Give one example of how the CNS interacts with the PNS to control a function in the body.
Phantom Limbs Explained, Plethrons, 2015
8.7 PSYCHOACTIVE DRUGS
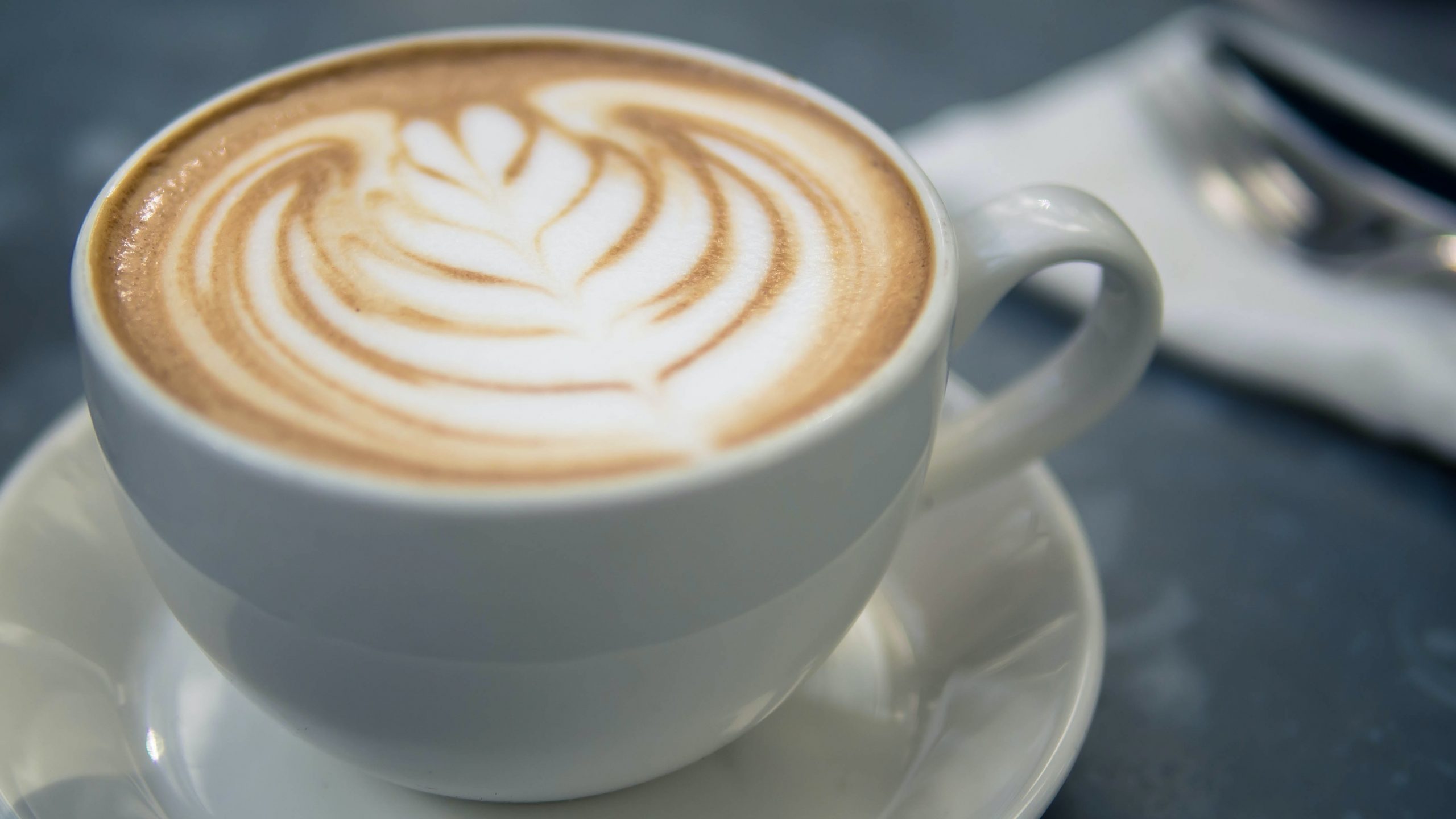
Art in a Cup
Who knew that a cup of coffee could also be a work of art? A talented barista can make coffee look as good as it tastes. If you are a coffee drinker, you probably know that coffee can also affect your mental state. It can make you more alert, and it may improve your concentration. That’s because the caffeine in coffee is a psychoactive drug. In fact, caffeine is the most widely consumed psychoactive substance in the world. In North America, for example, 90 per cent of adults consume caffeine daily.
WHAT ARE PSYCHOACTIVE DRUGS?
Psychoactive drugs are substances that change the function of the brain and result in alterations of mood, thinking, perception, and/or behavior. Psychoactive drugs may be used for many purposes, including therapeutic, ritual, or recreational purposes. Besides caffeine, other examples of psychoactive drugs include cocaine, LSD, alcohol, tobacco, codeine, and morphine. Psychoactive drugs may be legal prescription medications (codeine and morphine), legal nonprescription drugs (alcohol and tobacco), or illegal drugs (cocaine and LSD).
Cannabis (or marijuana) is also a psychoactive drug that while illegal in many countries is legal for use in Canada by individuals over the age of 19 years. Legal prescription medications (such as opioids) are also used illegally by increasingly large numbers of people. Some legal drugs, such as alcohol and nicotine, are readily available almost everywhere, as illustrated by the images below.
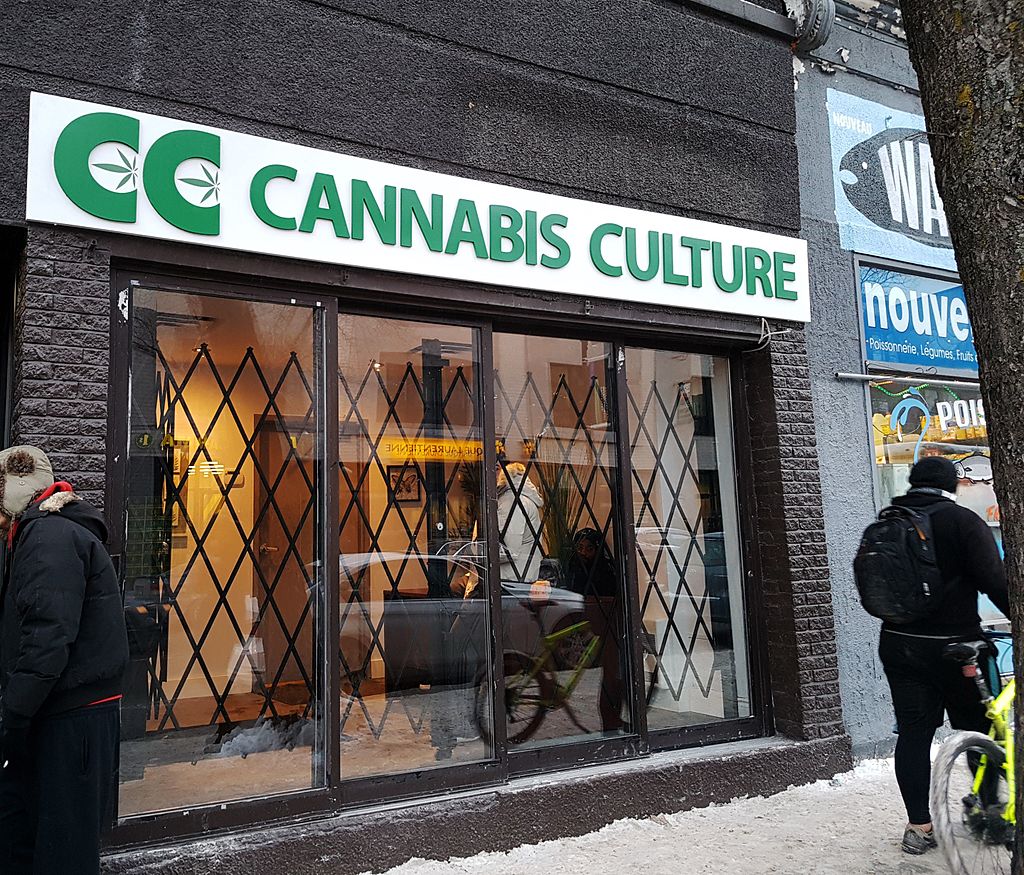
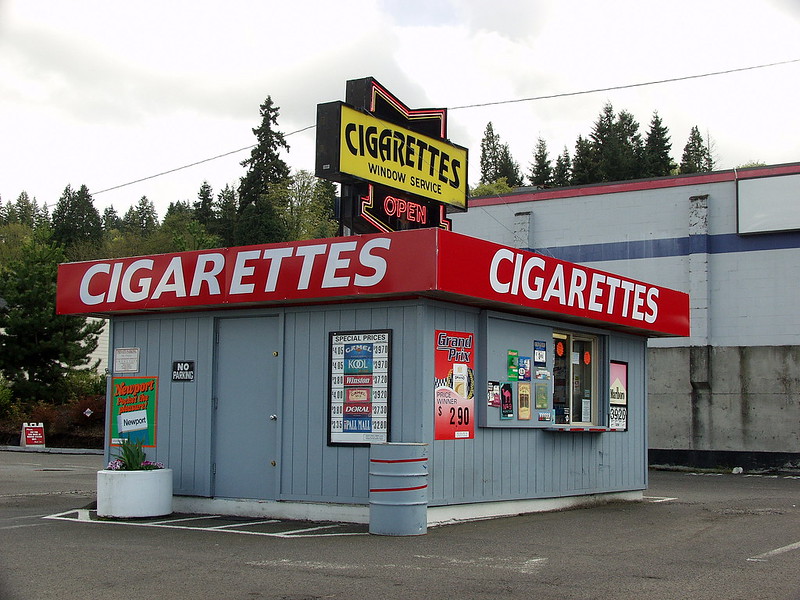
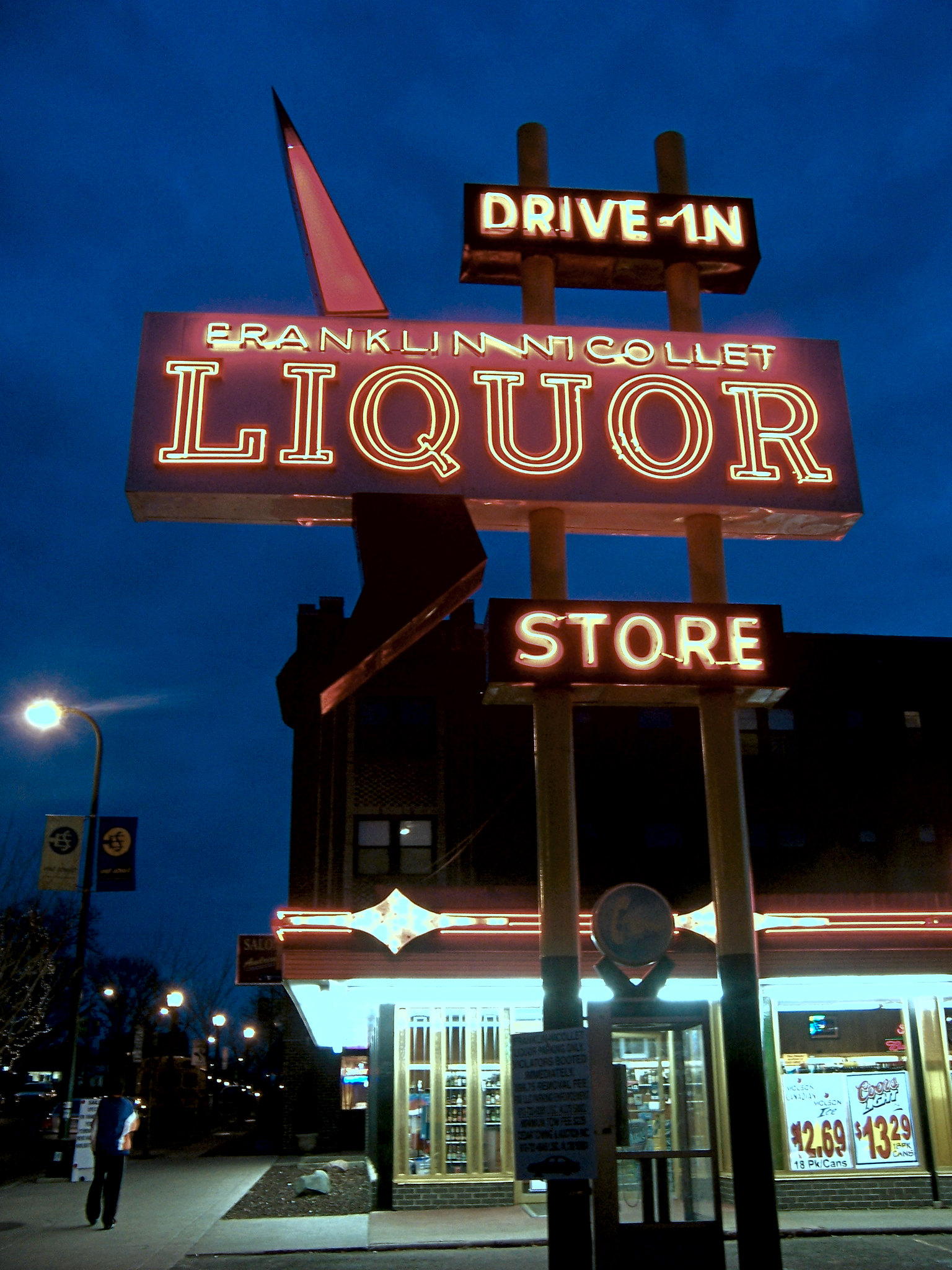
Figure 8.34 These psychoactive drugs are legal and accessible almost anywhere.
Classes of Psychoactive Drugs
Psychoactive drugs are divided into different classes based on their pharmacological effects. Several classes are listed below, along with examples of commonly used drugs in each class.
- Stimulants are drugs that stimulate the brain and increase alertness and wakefulness. Examples of stimulants include caffeine, nicotine, cocaine, and amphetamines (such as Adderall).
- Depressants are drugs that calm the brain, reduce anxious feelings, and induce sleepiness. Examples of depressants include ethanol (in alcoholic beverages) and opioids, such as codeine and heroin.
- Anxiolytics are drugs that have a tranquilizing effect and inhibit anxiety. Examples of anxiolytic drugs include benzodiazepines (such as diazepam/Valium), barbiturates (such as phenobarbital), opioids, and antidepressant drugs (such as sertraline/Zoloft).
- Euphoriants are drugs that bring about a state of euphoria, or intense feelings of well-being and happiness. Examples of euphoriants include the so-called “club drug” MDMA (ecstasy), amphetamines, ethanol, and opioids (such as morphine).
- Hallucinogens are drugs that can cause hallucinations and other perceptual anomalies. They also cause subjective changes in thoughts, emotions, and consciousness. Examples of hallucinogens include LSD, mescaline, nitrous oxide, and psilocybin.
- Empathogens are drugs that produce feelings of empathy, or sympathy with other people. Examples of empathogens include amphetamines and MDMA.
Many psychoactive drugs have multiple effects, so they may be placed in more than one class. One example is MDMA, pictured below, which may act both as a euphoriant and as an empathogen. In some people, MDMA may also have stimulant or hallucinogenic effects. As of 2016, MDMA had no accepted medical uses, but it was undergoing testing for use in the treatment of post-traumatic stress disorder and certain other types of anxiety disorders.
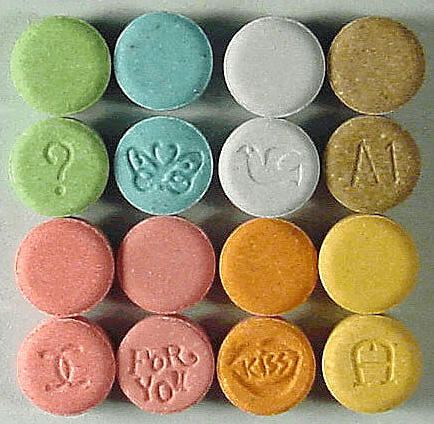
Mechanisms of Action
Psychoactive drugs generally produce their effects by affecting brain chemistry, which in turn may cause changes in a person’s mood, thinking, perception, and behavior. Each drug tends to have a specific action on one or more neurotransmitters or neurotransmitter receptors in the brain. Generally, they act as either agonists or antagonists.
- Agonists are drugs that increase the activity of particular neurotransmitters. They might act by promoting the synthesis of the neurotransmitters, reducing their reuptake from synapses, or mimicking their action by binding to receptors for the neurotransmitters.
- Antagonists are drugs that decrease the activity of particular neurotransmitters. They might act by interfering with the synthesis of the neurotransmitters or by blocking their receptors so the neurotransmitters cannot bind to them.
Consider the example of the neurotransmitter GABA. This is one of the most common neurotransmitters in the brain, and it normally has an inhibitory effect on cells. GABA agonists — which increase its activity — include ethanol, barbiturates, and benzodiazepines, among other psychoactive drugs. All of these drugs work by promoting the activity of GABA receptors in the brain.
USES OF PSYCHOACTIVE DRUGS
You may have been prescribed psychoactive drugs by your doctor. For example, your doctor may have prescribed you an opioid drug, such as codeine for pain (most likely in the form of Tylenol with added codeine). Chances are you also use nonprescription psychoactive drugs (like caffeine) for mental alertness. These are just two of the many possible uses of psychoactive drugs.
MEDICAL USES
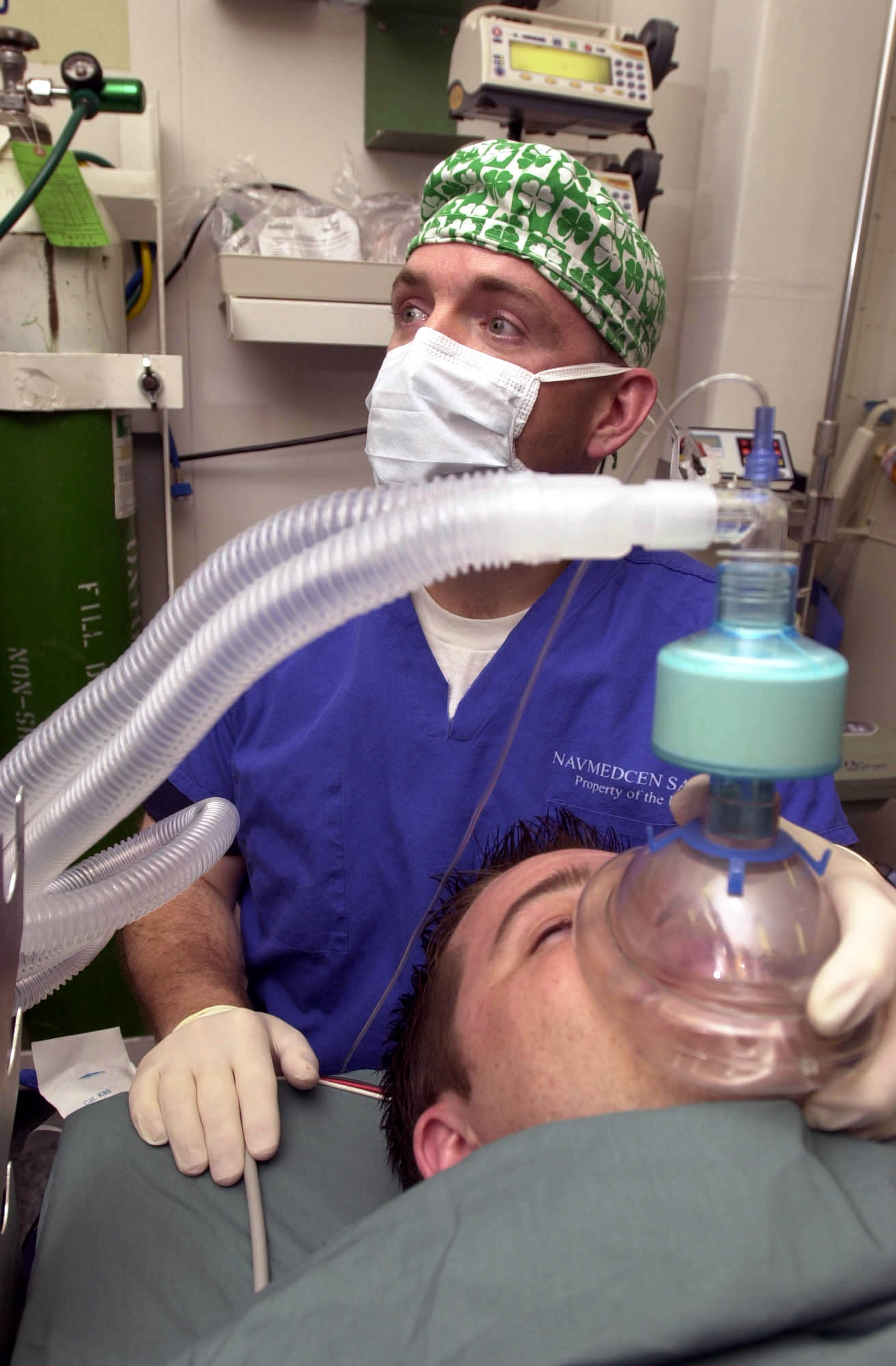
General anesthesia is one use of psychoactive drugs in medicine. With general anesthesia, pain is blocked and unconsciousness is induced. General anesthetics are most often used during surgical procedures and may be administered in gaseous form, as in Figure 8.36. General anesthetics include the drugs halothane and ketamine. Other psychoactive drugs are used to manage pain without affecting consciousness. They may be prescribed either for acute pain in cases of trauma (such as broken bones) or for chronic pain caused by arthritis, cancer, or fibromyalgia. Most often, the drugs used for pain control are opioids, such as morphine and codeine.
Many psychiatric disorders are also managed with psychoactive drugs. Antidepressants like sertraline, for example, are used to treat depression, anxiety, and eating disorders. Anxiety disorders may also be treated with anxiolytics, such as buspirone and diazepam. Stimulants (such as amphetamines) are used to treat attention deficit disorder. Antipsychotics (such as clozapine and risperidone) — as well as mood stabilizers, such as lithium — are used to treat schizophrenia and bipolar disorder. psychoactive drugs — including jimsonweed, psilocybin mushrooms, and cannabis — have also been used for millennia, by various peoples, for ritual purposes.
RITUAL USES
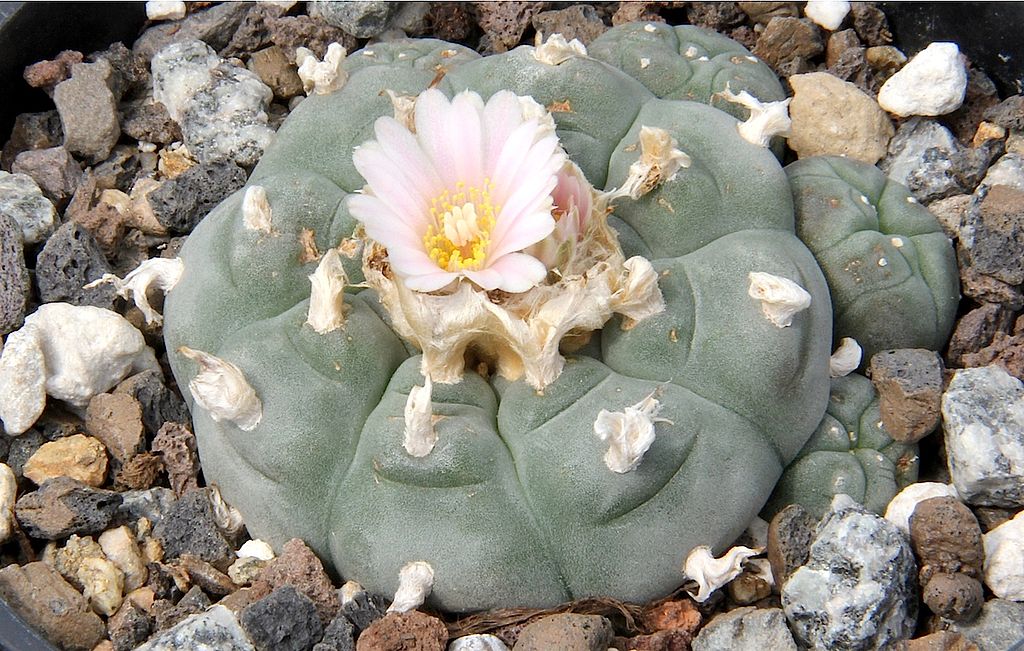
Certain psychoactive drugs, particularly hallucinogens, have been used for ritual purposes since prehistoric times. For example, Native Americans have used the mescaline-containing peyote cactus (pictured in Figure 8.37) for religious ceremonies for as long as 5,700 years. In prehistoric Europe, the mushroom Amanita muscaria, which contains a hallucinogenic drug called muscimol, was used for similar purposes.
Recreational Uses
The recreational use of psychoactive drugs generally has the purpose of altering one’s consciousness and creating a feeling of euphoria commonly called a “high.” Some of the drugs used most commonly for recreational purposes are cannabis, ethanol (alcohol), opioids, and stimulants (such as nicotine). Hallucinogens are also used recreationally, primarily for the alterations they cause in thinking and perception.
Some investigators have suggested that the urge to alter one’s state of consciousness is a universal human drive, similar to the drive to satiate thirst, hunger, or sexual desire. They think that this instinct is even present in children, who may attain an altered state by repetitive motions, such as spinning or swinging. Some nonhuman animals also exhibit a drive to experience altered states. They may consume fermented berries or fruit and become intoxicated. The way cats respond to catnip (see Figure 8.38) is another example.
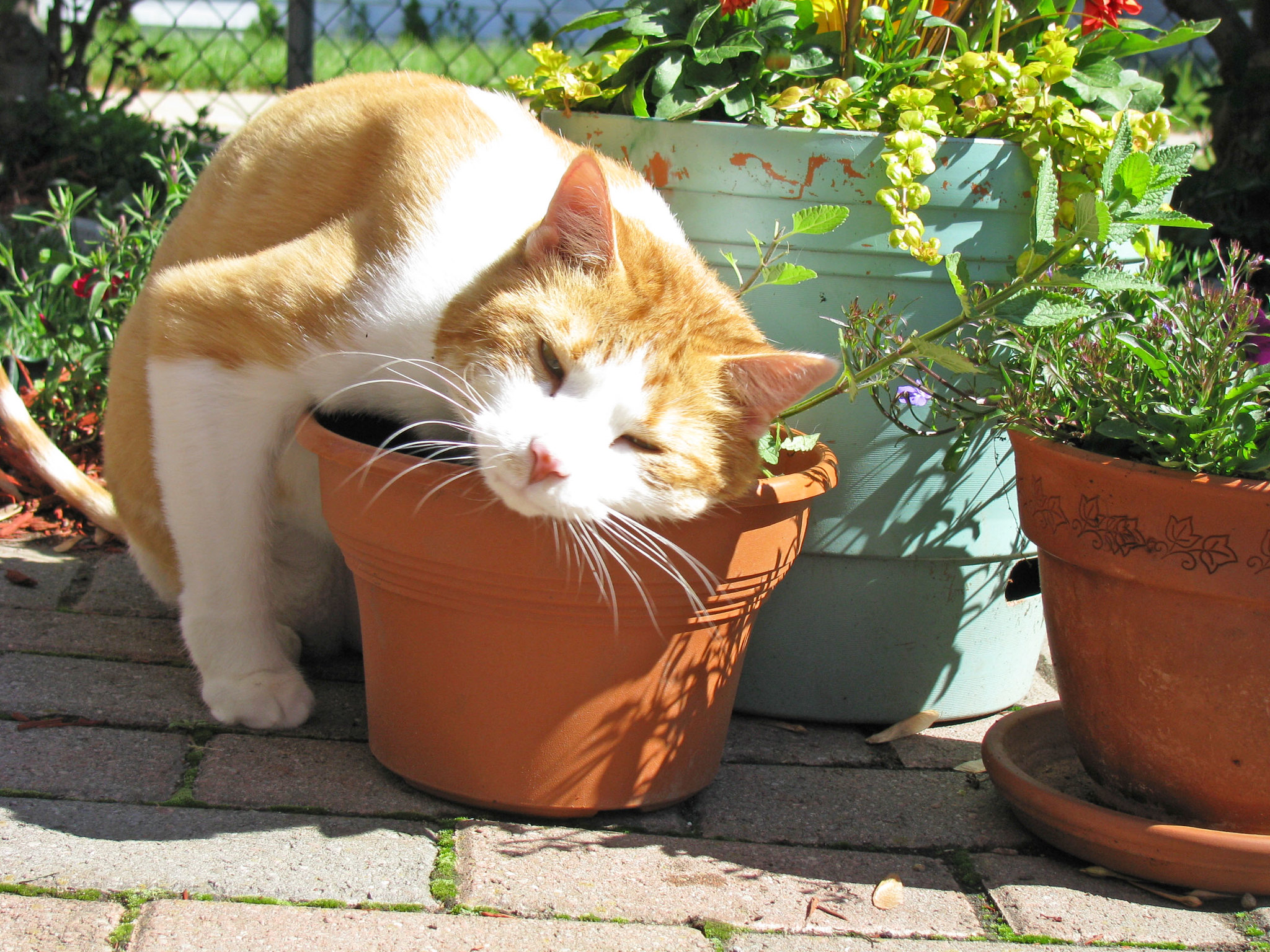
Addiction, Dependence, and Rehabilitation
Psychoactive substances often bring about subjective changes that the user may find pleasant (euphoria) or advantageous (increased alertness). These changes are rewarding and positively reinforcing, so they have the potential for misuse, addiction, and dependence . Addiction refers to the compulsive use of a drug, despite negative consequences that such use may entail. Sustained use of an addictive drug may produce dependence on the drug. Dependence may be physical and/or psychological. It occurs when cessation of drug use produces withdrawal symptoms. Physical dependence produces physical withdrawal symptoms, which may include tremors, pain, seizures, or insomnia. Psychological dependence produces psychological withdrawal symptoms, such as anxiety, depression, paranoia, or hallucinations.
Rehabilitation for drug dependence and addiction typically involves psychotherapy, which may include both individual and group therapy. Organizations such as Alcoholics Anonymous (AA) and Narcotics Anonymous (NA) may also be helpful for people trying to recover from addiction. These groups are self-described as international mutual aid fellowships, and their primary purpose is to help addicts achieve and maintain sobriety. In some cases, rehabilitation is aided by the temporary use of psychoactive substances that reduce cravings and withdrawal symptoms without creating addiction themselves. The drug methadone, for example, is commonly used to treat heroin addiction.
FEATURE: HUMAN BIOLOGY IN THE NEWS
In North America, a lot of media attention is currently given to a rising tide of opioid addiction and overdose deaths. Opioids are drugs derived from the opium poppy or synthetic versions of such drugs. They include the illegal drug heroin, as well as prescription painkillers such as codeine, morphine, hydrocodone, oxycodone, and fentanyl. In 2016, fentanyl received wide media attention when it was announced that an accidental fentanyl overdose was responsible for the death of music icon Prince. Fentanyl is an extremely strong and dangerous drug, said to be 50 to 100 times stronger than morphine, making risk of overdose death from fentanyl very high.
Consider the following facts:
- The number of prescriptions written for opioids quadrupled between 1999 and 2010. If you have been prescribed codeine, fentanyl, morphine, oxycodone, hydromorphone or medical heroin, then you have been prescribed an opiate.
- There are many long-term health effects of using opioids, which include:
- Increased tolerance to the drug.
- Liver damage.
- Substance use disorder or addiction.
Doctors, public health professionals, and politicians have all called for new policies, funding, programs, and laws to address the opioid epidemic. Changes that have already been made include a shift from criminalizing to medicalizing the problem, more treatment programs, and more widespread distribution and use of the opioid-overdose antidote naloxone (Narcan). Opioids can slow or stop a person’s breathing, which is what usually causes overdose deaths. Naloxone helps the person wake up and keeps them breathing until emergency medical treatment can be provided.
What, if anything, will work to stop the opioid epidemic in Canada and the United States? Keep watching the news to find out.
Review
- What are psychoactive drugs?
- Identify six classes of psychoactive drugs, along with an example of a drug in each class.
- Compare and contrast psychoactive drugs that are agonists and psychoactive drugs that are antagonists.
- Describe two medical uses of psychoactive drugs.
- Give an example of a ritual use of a psychoactive drug.
- Generally speaking, why do people use psychoactive drugs recreationally?
- Define addiction.
- Identify possible withdrawal symptoms associated with physical dependence on a psychoactive drug.
- Why might a person with a heroin addiction be prescribed the psychoactive drug methadone?
- The prescription drug Prozac inhibits the reuptake of the neurotransmitter serotonin, causing more serotonin to be present in the synapse. Prozac can elevate mood, which is why it is sometimes used to treat depression. Answer the following questions about Prozac:
- Is Prozac an agonist or an antagonist for serotonin? Explain your answer.
- Is Prozac a psychoactive drug? Explain your answer.
- Name three classes of psychoactive drugs that include opioids.
- True or False: All psychoactive drugs are either illegal or available by prescription only.
- True or False: Anxiolytics might be prescribed by a physician.
- Name two drugs that activate receptors for the neurotransmitter GABA. Why do you think these drugs generally have a depressant effect?
How does caffeine keep us awake? – Hanan Qasim, TED-Ed, 2017.
How do drugs affect the brain? – Sara Garofalo, TED-Ed, 2017.
8.8 Memory
Why is memory important to the human body?
Memory is an information processing system that we often compare to a computer. Memory is the set of processes used to encode, store, and retrieve information over different periods of time. It is essential for learning, allows us to reflect upon our past, and is part of our consciousness.
Figure 8.39 Encoding involves the input of information into the memory system. Storage is the retention of the encoded information. Retrieval, or getting the information out of memory and back into awareness, is the third function.
ENCODING
We get information into our brains through a process called encoding, which is the input of information into the memory system. Once we receive sensory information from the environment, our brains label or code it. We organize the information with other similar information and connect new concepts to existing concepts. Encoding information occurs through both automatic processing and effortful processing.
When you first learn new skills such as driving a car, you have to put forth effort and attention to encode information about how to start a car, how to brake, how to handle a turn, and so on. Once you know how to drive, you can encode additional information about this skill automatically.
STORAGE
Once the information has been encoded, we have to retain it. Our brains take the encoded information and place it in storage. Storage is the creation of a permanent record of information.
In order for a memory to go into storage (i.e., long-term memory), it has to pass through two distinct stages: Short-Term Memory and Long-Term Memory.
Short-Term Memory
Short-term memory is a temporary storage system involving the Limbic System that processes incoming sensory memory; sometimes it is called working memory. Short-term memory storage lasts about 20 seconds. Think of short-term memory as the information you have displayed on your computer screen—a document, a spreadsheet, or a web page. Information in short-term memory either goes to long-term memory (when you save it to your hard drive) or it is discarded (when you delete a document or close a web browser).
Short=term memory involves temporary changes in the function of synapses where action potentials occur quickly.
You may find yourself asking, “How much information can our memory handle at once?” Research on the capacity of memory found that most people can retain about 7 items in short-term memory. Some remember 5, some 9, so he called the capacity of short-term memory the range of 7 items plus or minus 2. To explore the capacity and duration of your short-term memory, have a partner read the strings of random numbers below out loud to you, beginning each string by saying, “Ready?” and ending each by saying, “Recall,” at which point you should try to write down the string of numbers from memory. Note the longest string at which you got the series correct.
Figure 8.40 Work through this series of numbers using the recall exercise explained above to determine the longest string of digits that you can store.
Long-term Memory
Long-term memory is the continuous storage of information into the cerebral cortex. This type of memory involves permanent changes to the neurons –
- Dendrites branch and elongate
- Increase in the number of synaptic terminals
- Increase in the number of neurotransmitters released
Unlike short-term memory, the storage capacity of long-term memory has no limits. It encompasses all the things you can remember that happened more than just a few minutes ago to all of the things that you can remember that happened days, weeks, and years ago. In keeping with the computer analogy, the information in your long-term memory would be like the information you have saved on the hard drive. It isn’t there on your desktop (your short-term memory), but you can pull up this information when you want it, at least most of the time. Not all long-term memories are strong memories. Some memories can only be recalled through prompts. For example, you might easily recall a fact— “What is the capital of the United States?”—or a procedure— “How do you ride a bike?”—but you might struggle to recall the name of the restaurant you had dinner when you were on vacation in France last summer. A prompt, such as that the restaurant was named after its owner, who spoke to you about your shared interest in soccer, may help you recall the name of the restaurant.
Strengthening Your Memory
We’ve discussed the importance of zeroing in on the main concepts you learn in class and of transferring them from short-term to long-term memory. But how can you work to strengthen your overall memory? Some people have stronger memories than others, but memorizing new information takes work for anyone. Below are some strategies that can aid memory.
Rehearsal
One strategy is rehearsal, or the conscious repetition of information to be remembered. This strategy is linked to studying material frequently for shorter periods of time. You may not remember when or how you learned skills like riding a bike or tying your shoes. Mastery came with practice, and at some point, the skills became second nature. Academic learning is no different: if you spend enough time with important course concepts and practice them often, you will know them in the same way you know how to ride a bike, almost without thinking about them. For example, think about how you learned your multiplication tables. You may recall that 6 x 6 = 36, 6 x 7 = 42, and 6 x 8 = 48. Memorizing these facts is rehearsal.
Incorporate visuals
Visual aids like note cards, concept maps, and highlighted text are ways of making information stand out. Because they are shorter and more concise, they have the advantage of making the information to be memorized seem more manageable and less daunting (than an entire textbook chapter, for example). Some students write key terms on note cards and hang them around their desk or mirror so that they routinely see them and study them without even trying.
Create mnemonics
Memory devices known as mnemonics can help you retain information while only needing to remember a unique phrase or letter pattern that stands out. Mnemonic devices are memory aids that help us organize information for encoding. They are especially useful when we want to recall larger bits of information such as steps, stages, phases, and parts of a system.
There are different types of mnemonic devices, such as the acronym. An acronym is a word formed by the first letter of each of the words you want to remember. For example, even if you live near one, you might have difficulty recalling the names of all five Great Lakes. What if I told you to think of the word Homes? HOMES is an acronym that represents Huron, Ontario, Michigan, Erie, and Superior: the five Great Lakes.
Another type of mnemonic device is an acrostic: you make a phrase of all the first letters of the words. For example, if you are taking a math test and you are having difficulty remembering the order of operations, recalling the sentence “Please Excuse My Dear Aunt Sally” will help you, because the order of mathematical operations is Parentheses, Exponents, Multiplication, Division, Addition, Subtraction. There also are jingles, which are rhyming tunes that contain keywords related to the concept, such as “i before e, except after c.”
You might use a mnemonic device to help you remember someone’s name, a mathematical formula, or the six levels of Bloom’s taxonomy.
Figure 8.39 This is a knuckle mnemonic to help you remember the number of days in each month. Months with 31 days are represented by the protruding knuckles and shorter months fall in the spots between knuckles. (credit: modification of work by Cory Zanker)
Chunking
Another strategy is chunking, where you organize information into manageable bits or chunks. Chunking is useful when trying to remember information like dates and phone numbers. Instead of trying to remember 5205550467, you remember the number as 520-555-0467. So, if you met an interesting person at a party and you wanted to remember his phone number, you would naturally chunk it, and you could repeat the number over and over, combining the strategies of chunking and rehearsal.
Connect new information to old information
Take stock of what you already know—information that’s already stored in long-term memory—and use it as a foundation for learning newer information. It’s easier to remember new information if you can connect it to old information or to a familiar frame of reference. For example, if you are taking a sociology class and are learning about different types of social groups, you may be able to think of examples from your own experiences that relate to the different types.
Get quality sleep
Although some people require more or less sleep than the recommended amount, most people should aim for six–eight hours every night. School puts a lot of demands on the brain, and, like tired muscles after a long workout, your brain needs to rest after being exercised and taking in all sorts of new information during the day. Plus, while you are sleeping, your brain is still at work. During sleep the brain organizes and consolidates information to be stored in long-term memory. A good night’s rest can help you remember more and feel prepared for learning the next day.
Memory also relies on effective studying behaviors, like choosing where you study, how you study, and with whom you study. The following video provides specific studying strategies that can improve your memory.
“Studying Advice: Tips for College Students” StudentMentor.org’s Student Video Blog Series
CASE STUDY: FADING MEMORY
Although we are all forgetful sometimes, most people do not have trouble remembering things that are important or routine to them, such as a friend’s name or how to get to class. Our brain — the control center of the nervous system and the rest of the body — normally allows us to retain and recall information. If, however, the brain is damaged, a person may need to rely excessively on external reminders — like this wall of sticky notes — rather than their own memory… if they can remember to write things down in the first place.
One person having trouble with her memory is 68-year-old Rosa. Rosa has been struggling to remember where she has set down objects in her house, and she forgot about a few doctor’s appointments and lunches she planned with friends. Her family began to notice that she would sometimes fail to recall recent conversations, requiring them to repeat things to her. Rosa would also sometimes struggle to find the right word in a conversation, and would put objects in unusual places, such as the milk in a cabinet instead of the refrigerator. While most people do things like this occasionally, it seemed to Rosa and her family that it was happening to her more regularly.
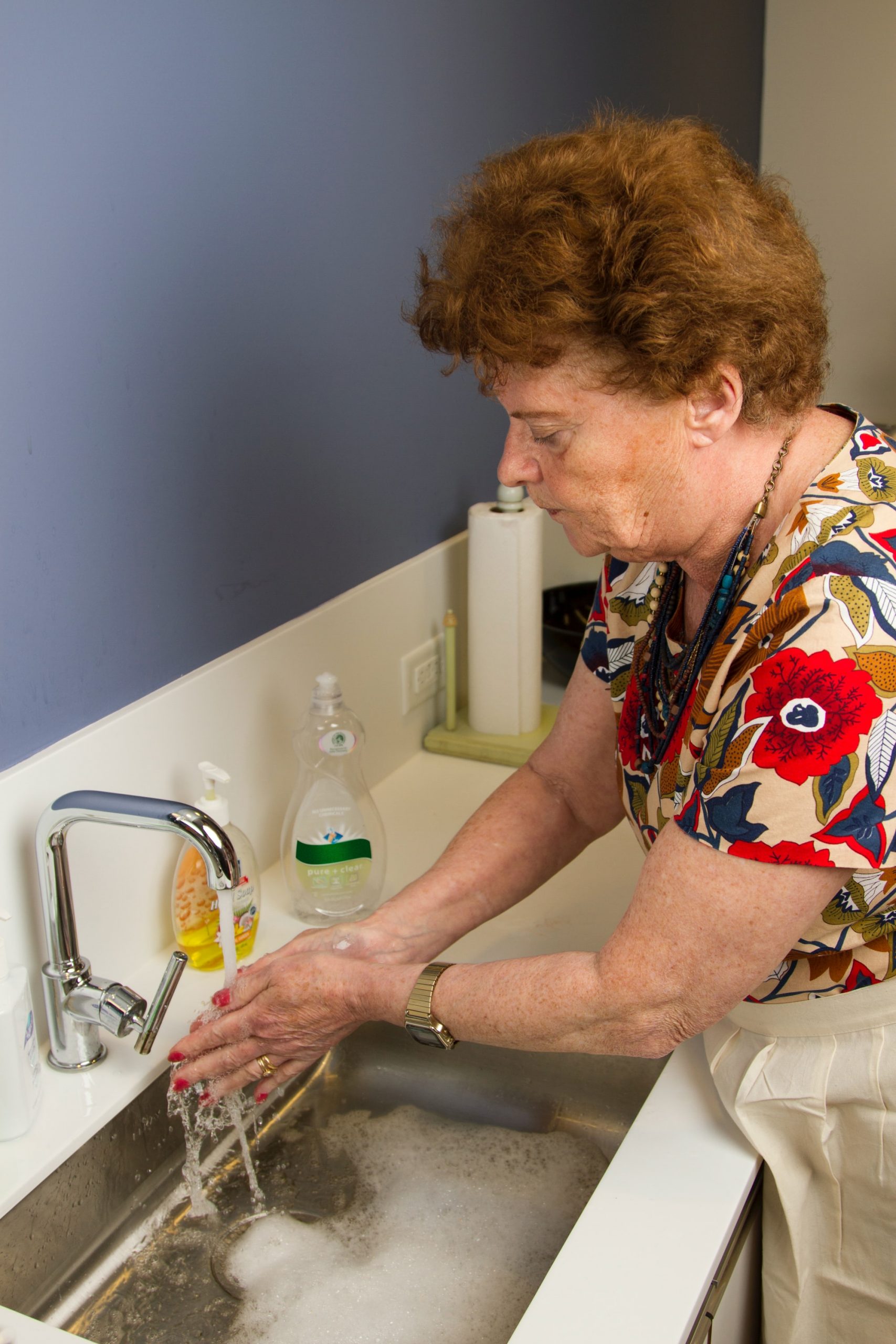
Rosa also had other symptoms that were impacting her life, such as having trouble paying her bills on time and managing her budget, which she had previously done well. She ascribed these lapses in memory and mental functioning to the normal effects of aging, but her family was concerned. They noticed that she was also more irritable than usual and would sometimes verbally lash out at them, which was not like her. When she became disoriented on a walk around her neighborhood and a neighbor had to escort her home, her family convinced her to see a doctor.
Besides a complete physical exam and lab tests, Rosa’s doctor interviewed Rosa and her family about her memory, ability to carry out daily tasks, and mood changes. He also administered a variety of tests to assess her memory and cognitive functioning, such as her ability to solve problems and use numbers and language correctly. Finally, he ordered a scan of her brain to investigate whether a tumor or some other observable cause was leading to changes in the functioning of her brain.
Based on the results of these tests, Rosa’s doctor concluded that she most likely has mild Alzheimer’s disease (AD). AD results from abnormal changes in the molecules and cells of the brain, characterized by clumps of proteins (called amyloid plaques) between brain cells and tangled bundles of protein fibres (called neurofibrillary tangles) within certain brain cells. The affected brain cells stop functioning properly, lose their connections to other brain cells, and will eventually die. Figure 8.41 shows part of a cross-section of a brain from a patient who had severe AD, compared to a similar cross-section of a healthy brain. You can see how severely shrunken the brain with AD is, due to the death of many brain cells.
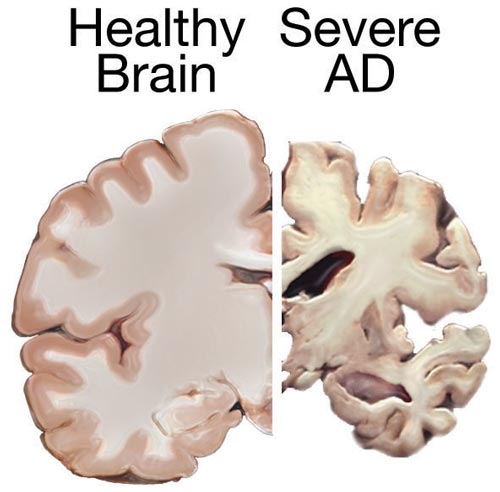
AD is a progressive disease, which means the damage and associated symptoms get worse over time. Clinicians have categorized the progression into three main stages — mild, moderate, and severe AD. Typically, AD cannot be definitively diagnosed until after death, when the brain tissue can be directly examined for plaques and tangles. Based on Rosa’s symptoms and the results of her tests, though, her doctor thinks she most likely has mild AD. At this stage, the brain has started changing, but resulting symptoms are not yet severe.
Although there is currently no cure for AD and Rosa will eventually get worse, her doctor says that medications and behavioral therapies may improve and prolong her functioning and quality of life over the next few years. He prescribes a medication that improves communication between brain cells, which has been shown to help some people with AD.
Think about the following questions:
- Based on Rosa’s symptoms, which parts of her brain may have been affected by Alzheimer’s disease?
- How are messages sent between cells in the nervous system? What molecules are involved in this process? In what ways can drugs alter this process?
- Why can’t Rosa’s brain simply grow new cells to replace the ones that have died?
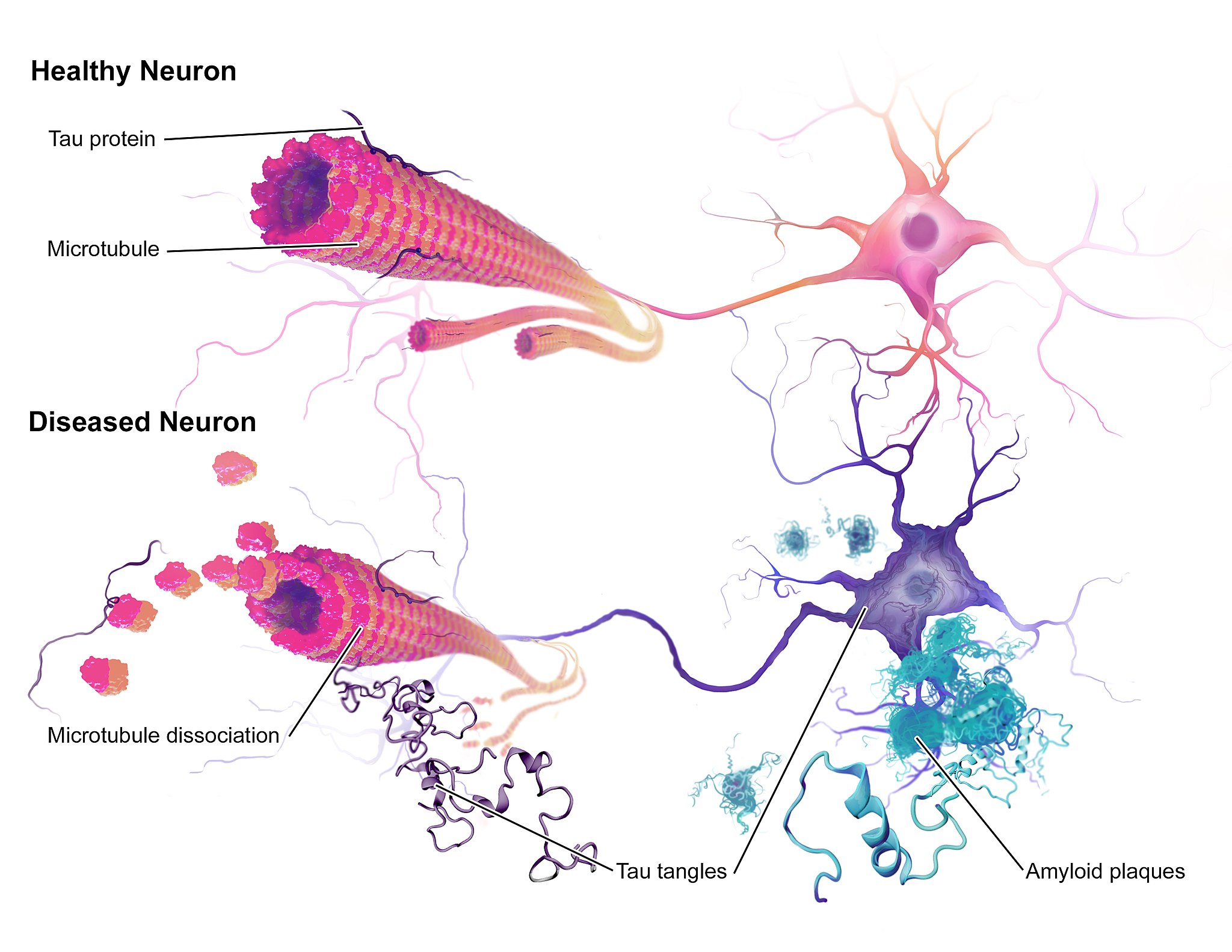
The illustration above (Figure 8.42) shows some of the molecular and cellular changes that occur in Alzheimer’s disease (AD). Rosa was diagnosed with AD at the beginning of this chapter after experiencing memory problems and other changes in her cognitive functioning, mood, and personality. These abnormal changes in the brain include the development of amyloid plaques between brain cells and neurofibrillary tangles inside of neurons. These hallmark characteristics of AD are associated with the loss of synapses between neurons, and ultimately the death of neurons.
After reading this chapter, you should have a good appreciation for the importance of keeping neurons alive and communicating with each other at synapses. The nervous system coordinates all of the body’s voluntary and involuntary activities. It interprets information from the outside world through sensory systems, and makes appropriate responses through the motor system, through communication between the PNS and CNS. The brain directs the rest of the nervous system and controls everything from basic vital functions (such as heart rate and breathing) to high-level functions (such as problem solving and abstract thought). The nervous system can perform these important functions by generating action potentials in neurons in response to stimulation and sending messages between cells at synapses, typically using chemical neurotransmitter molecules. When neurons are not functioning properly, lose their synapses, or die, they cannot carry out the signaling essential for the proper functioning of the nervous system.
AD is a progressive neurodegenerative disease, meaning that the damage to the brain becomes more extensive as time goes on. The picture in Figure 8.43 illustrates how the damage progresses from before AD is diagnosed (preclinical AD), to mild and moderate AD, to severe AD.
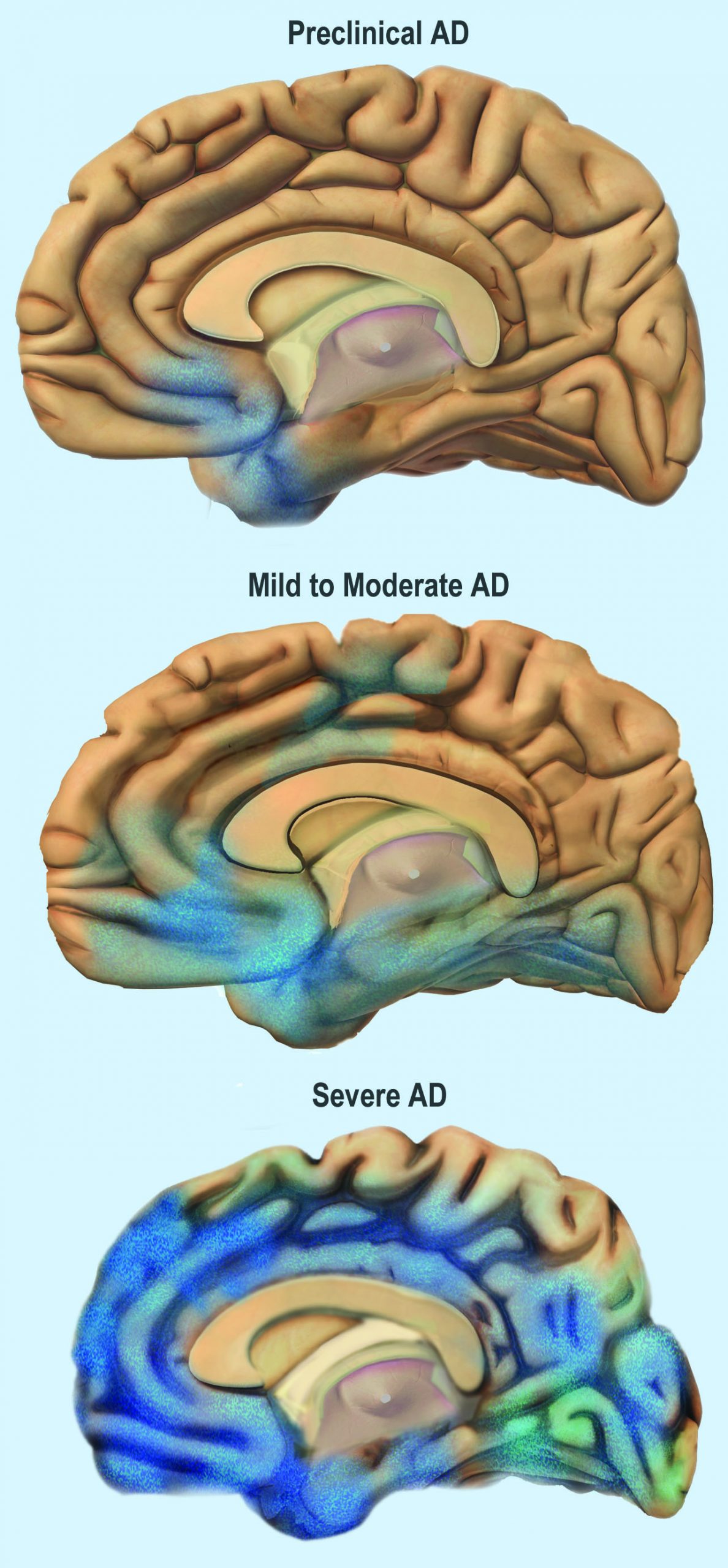
You can see that the damage starts in a relatively small location toward the bottom of the brain. One of the earliest brain areas to be affected by AD is the hippocampus. As you have learned, the hippocampus is important for learning and memory, which explains why many of Rosa’s symptoms of mild AD involve deficits in memory, such as trouble remembering where she placed objects, recent conversations, and appointments.
As AD progresses, more of the brain is affected, including areas involved in emotional regulation, social behavior, planning, language, spatial navigation, and higher-level thought. Rosa is beginning to show signs of problems in these areas, including irritability, lashing out at family members, getting lost in her neighborhood, problems finding the right words, putting objects in unusual locations, and difficulty in managing her finances. You can see that as AD progresses, damage spreads further across the cerebrum, which you now know controls conscious functions like reasoning, language, and interpretation of sensory stimuli. You can also see how the frontal lobe — which controls executive functions such as planning, self-control, and abstract thought — becomes increasingly damaged.
Increasing damage to the brain causes corresponding deficits in functioning. In moderate AD, patients have increased memory, language, and cognitive deficits, compared to mild AD. They may not recognize their own family members, and may wander and get lost, engage in inappropriate behaviors, become easily agitated, and have trouble carrying out daily activities such as dressing. In severe AD, much of the brain is affected. Patients usually cannot recognize family members or communicate, and they are often fully dependent on others for their care. They begin to lose the ability to control their basic functions, such as bladder control, bowel control, and proper swallowing. Eventually, AD causes death, usually as a result of this loss of basic functions.
For now, Rosa only has mild AD and is still able to function relatively well with care from her family. The medication her doctor gave her has helped improve some of her symptoms. It is a cholinesterase inhibitor, which blocks an enzyme that normally degrades the neurotransmitter acetylcholine. With more of the neurotransmitter available, more of it can bind to neurotransmitter receptors on postsynaptic cells. Therefore, this drug acts as an agonist for acetylcholine, which enhances communication between neurons in Rosa’s brain. This increase in neuronal communication can help restore some of the functions lost in early Alzheimer’s disease and may slow the progression of symptoms.
But medication such as this is only a short-term measure, and does not halt the progression of the underlying disease. Ideally, the damaged or dead neurons would be replaced by new, functioning neurons. Why does this not happen automatically in the body? As you have learned, neurogenesis is very limited in adult humans, so once neurons in the brain die, they are not normally replaced to any significant extent. Scientists, however, are studying the ways in which neurogenesis might be increased in cases of disease or injury to the brain. They are also investigating the possibility of using stem cell transplants to replace damaged or dead neurons with new neurons. But this research is in very early stages and is not currently a treatment for AD.
One promising area of research is in the development of methods to allow earlier detection and treatment of AD, given that the changes in the brain may actually start ten to 20 years before diagnosis of AD. A radiolabeled chemical called Pittsburgh Compound B (PiB) binds to amyloid plaques in the brain, and in the future, it may be used in conjunction with brain imaging techniques to detect early signs of AD. Scientists are also looking for biomarkers in bodily fluids (such as blood and cerebrospinal fluid) that might indicate the presence of AD before symptoms appear. Finally, researchers are also investigating possible early and subtle symptoms (such as changes in how people move or a loss of smell) to see whether they can be used to identify people who will go on to develop AD. This research is in the early stages, but the hope is that patients can be identified earlier, allowing for earlier and more effective treatment, as well as more planning time for families.
Scientists are also still trying to fully understand the causes of AD, which affects more than five million Americans. Some genetic mutations have been identified as contributors, but environmental factors also appear to be important. With more research into the causes and mechanisms of AD, hopefully a cure can be found, and people like Rosa can live a longer and better life.
Attributions
This chapter is composed of text taken from of the following sources:
Lucas, L. (2007-2024). Memory and information processing. Austin Community College. [CC BY-NC-SA 4.0]. Retrieved from [Learning Framework: Effective Strategies for College Success, Learning About Learning, Chapter 9: Memory and Information Processing | OER Commons]
Miller, C. (2020). Human biology: Human anatomy and physiology. Thompson Rivers University. [CC BY NC]. Retrieved from [Human Biology – Simple Book Publishing (tru.ca)]
Wakim, S., & Grewal, M. (2021). Nerve impulses. CK-12 License. Edited to the style and standards of the LibreTexts platform. Retrieved from https://www.ck12.org/book/ck-12-human-biology/