1 Introduction to Human Biology and the Scientific Method
Human biology is the scientific study of the human species. This chapter gives a basic understanding of the human body and how we fit into the natural world. It will also give you an understanding about how scientists think and how they “do” science. It describes how scientific theories develop and how scientists investigate questions to advance scientific knowledge. You’ll learn:
- The traits shared by all living thing
- Classification of living organisms
- The basic principles that underlie all of biology
- The vast diversity of living organisms
- What it means to be human and the characteristics that separate us from other living organisms
- Our place in the animal kingdom
- Organization of the human body
- The definition and process of science.
- The Scientific Method
1.1: Shared Traits of All Living Things
You’ve probably seen this famous statue created by the French sculptor Auguste Rodin. Rodin’s skill as a sculptor is evident because the statue looks so lifelike. In fact, the statue is made of rock so its only resemblance to life is how it appears. How does a statue made of rock differ from a living, breathing human being or other living organisms? What is life? What does it mean to be alive? Science has answers to these questions.

homeostasis
organization
metabolism
growth
adaptation
response to stimuli
reproduction
Homeostasis
All living things are able to maintain a more-or-less constant internal environment. They keep things relatively stable on the inside regardless of the conditions around them. The condition in which a system is maintained in a more-or-less steady state is called homeostasis. Human beings, for example, maintain a stable internal body temperature. If you go outside when the air temperature is below freezing, your body doesn’t freeze. Instead, by shivering and other means, it maintains a stable internal temperature.
Organization
Living things have multiple levels of organization. Their molecules are organized into one or more cells. A cell is the basic unit of the structure and function of living things. Cells are the building blocks of living organisms. An average adult human being, for example, consists of trillions of cells. Living things may appear very different from one another on the outside, but their cells are very similar. Compare the human cells and onion cells in the figure below. What similarities do you see?
Organisms may be composed one cell or many. One celled organisms known as unicellular have all the necessary components to maintain life. Multicellular organisms have more than one cell and may contain trillions of cells.

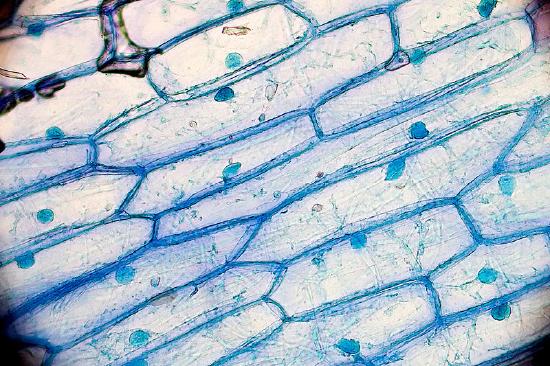
Metabolism
All living things can use energy. Their cells have the “machinery” of metabolism, which is the building up and breaking down of chemical compounds. Living things can transform energy by converting chemicals and energy into cellular components. This form of metabolism is called anabolism. They can also break down, or decompose, organic matter, which is called catabolism. Living things require energy to maintain internal conditions (homeostasis), for growth, and other life processes.
Growth
All living things have the capacity for growth. Growth is an increase in size that occurs when there is a higher rate of anabolism than catabolism. For example, a human infant has changed dramatically in size by the time it reaches adulthood, as is apparent from the image below. In what other ways do we change as we grow from infancy to adulthood?

Adaptations and Evolution
An adaptation is a characteristic of populations. Individuals of a population carry a variety of genes. When the environment changes, some individuals of the population can withstand the changed conditions and reproduce more than the individuals who cannot live in the given environment. A change in the allele frequencies and makeup of the populations over time is called evolution. It comes about through the process of natural selection.
Response to Stimuli
All living things detect changes in their environment and respond to them. A response can take many forms, from the movement of a unicellular organism in response to external chemicals (called chemotaxis), to complex reactions involving all the senses of a multicellular organism. A response is often expressed by motion; for example, the leaves of a plant turning toward the sun (called phototropism).
Reproduction
All living things are capable of reproduction. Reproduction is the process by which living things give rise to offspring. Reproduction may be as simple as a single cell dividing into two cells. This is how bacteria reproduce. Reproduction in human beings and many other organisms is much more complicated. Nonetheless, whether a living thing is a human being or a bacterium, it is normally capable of reproduction.
Review
- Identify seven traits that most scientists agree are shared by all living things.
- What is homeostasis? What is one-way humans fulfill this criterion of living things?
- Define reproduction and describe an example.
- What are the two types of metabolism described here and what are their differences?
- What are two processes that use energy in a living thing?
- Give an example of a response to stimuli in humans.
- Evolution occurs through ___________ ____________ .
1.2: Diversity of Life
The collage below shows six kingdoms into which all of Earth’s living things are commonly classified. How many species are there in each kingdom? In a word, millions. A total of almost 2 million living species have already been identified, and new species are being discovered all the time. Scientists estimate that there may be as many as 30 million different species alive on Earth today! Clearly, there is a tremendous variety of life on Earth.
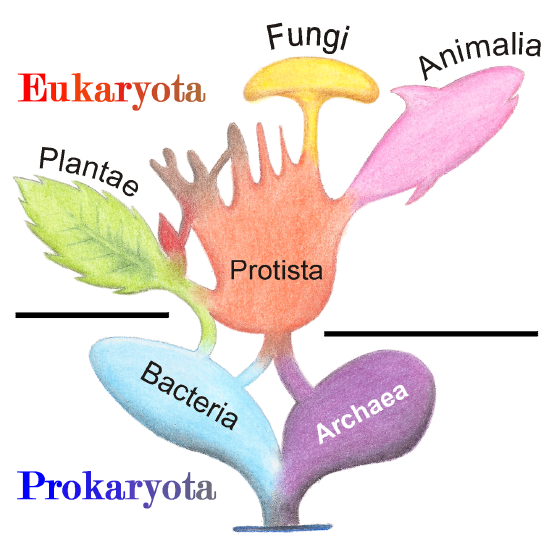
What Is Biodiversity?
Biological diversity, or biodiversity, refers to all of the variety of life that exists on Earth. Biodiversity can be described and measured at three different levels: species, genetic, and ecosystem diversity.
- Species diversity refers to the number of different species in an ecosystem or on Earth as a whole. This is the commonest way to measure biodiversity. Current estimates for Earth’s total number of living species range from 5 to 30 million species.
- Genetic diversity refers to the variation in genes within all these species.
- Ecosystem diversity refers to the variety of ecosystems on Earth. An ecosystem is a system formed by populations of many different species interacting with each other and their environment.
Defining a Species
Biodiversity is most often measured by counting species, but what is a species? The answer to that question is not as straightforward as you might think. The formal biological definition of species is a group of actually or potentially interbreeding organisms. This means that members of the same species are similar enough to each other to produce fertile offspring together. By this definition of species, all human beings alive today belong to one species, Homo sapiens. All humans can potentially interbreed with each other but not with members of any other species.
In the real world, it isn’t always possible to make the observations needed to determine whether different organisms can interbreed. For one thing, many species reproduce asexually, so individuals never interbreed even with members of their own species. When studying extinct species represented by fossils, it is usually impossible to know whether different organisms could interbreed. Therefore, in practice, many biologists and virtually all paleontologists generally define species on the basis of morphology, rather than breeding behavior. Morphology refers to the form and structure of organisms. For classification purposes, it generally refers to relatively obvious physical traits. Typically, the more similar to one another different organisms appear, the greater the chance that they will be classified in the same species.
Classifying Living Things
People have been trying to classify the tremendous diversity of life on Earth for more than two thousand years. The science of classifying organisms is called taxonomy. Classification is an important step in understanding the present diversity and past evolutionary history of life on Earth. It helps make sense of the overwhelming diversity of living things. Scientists classify organisms according to three criteria:
- Whether or not the cells of the organism contain a nucleus. Organisms whose cells do not contain a nucleus and few organelles are called Prokaryotes. Eukaryotes are organisms that have cells with a nucleus and true organelles.
- The number of cells that make up the organism. Organisms consisting of only one cell such as bacteria and protozoans are unicellular. Those with more than one cell are known as multicellular organisms.
- How organisms obtain their nutrients.
Linnaean Classification
All modern classification systems have their roots in the Linnaean classification system. It was developed by Swedish botanist Carolus Linnaeus in the 1700s. He tried to classify all living things that were known at his time. He grouped together organisms that shared obvious morphological traits, such as the number of legs or shape of leaves.
The Linnaean system of classification consists of a hierarchy of groupings, called taxa (singular, taxon). Figure 1.5 shows an expanded version of Linnaeus’s original classification system. In the original system, taxa range from the kingdom to the species. The kingdom is the largest and most inclusive grouping. It consists of organisms that share just a few basic similarities. There are four kingdoms: plant, animal fungi and protists. The species is the smallest and most exclusive grouping. Ideally, it consists of organisms that are similar enough to interbreed, as discussed above. Similar species are classified together in the same genus (plural, genera), similar genera are classified together in the same family, and so on all the way up to the kingdom.
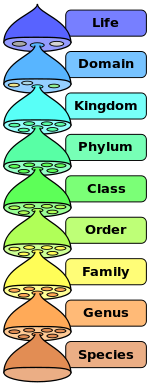
Binomial Nomenclature
Perhaps the single greatest contribution Linnaeus made to science was his method of naming species. This method, called binomial nomenclature, gives each species a unique, two-word Latin name consisting of the genus name followed by a specific species identifier. An example is Homo sapiens, the two-word Latin name for humans. It literally means “wise human.” This is a reference to our big brains.
Why is having two names so important? It is similar to people having a first and a last name. You may know several people with the first name Michael, but adding Michael’s last name usually pins down exactly who you mean. In the same way, having two names uniquely identifies a species.
Revisions in the Linnaean Classification
Linnaeus published his classification system in the 1700s. Since then, many new species have been discovered. Scientists can also now classify organisms on the basis of their biochemical and genetic similarities and differences rather than just their outward morphology. These changes have led to revisions in the original Linnaean system of classification.
A major change to the Linnaean system is the addition of a new taxon called the domain. The domain is a taxon that is larger and more inclusive than the kingdom. Most biologists agree that there are three domains of life on Earth: Bacteria, Archaea, and Eukarya (Figure 1.6). Both the Bacteria and the Archaea domains consist of single-celled organisms that lack a nucleus. This means that their genetic material is not enclosed within a membrane inside the cell. The Eukarya domain, in contrast, consists of all organisms whose cells have a nucleus. In other words, their genetic material is enclosed within a membrane inside the cell. The Eukarya domain is made up of both single-celled and multicellular organisms. This domain includes several kingdoms, including the animal, plant, fungus, and protist kingdoms.
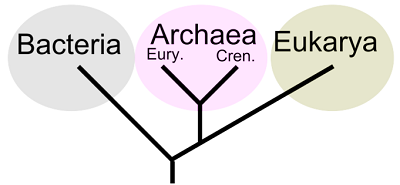
Phylogenetic Classification
Linnaeus classified organisms based on morphology. Basically, organisms were grouped together if they looked alike. After Darwin published his theory of evolution in the 1800s, scientists looked for a way to classify organisms that took into account phylogeny. Phylogeny is the evolutionary history of a group of related organisms. It is represented by a phylogenetic tree, or some other tree-like diagram, like the one in Figure 1.6 for the three domains. A phylogenetic tree shows how closely related different groups of organisms are to one another. Each branching point represents a common ancestor of the branching groups. Figure 1.6, for example, shows that the Eukarya shared a more recent common ancestor with the Archaea than they did with the Bacteria. This is based on comparisons of important similarities and differences between the three domains.
How are humans different from other species?
Humans are by no means the largest or fastest species on the planet, however, there are certain features that set us apart from other organisms.
- Bipedalism – Humans stand upright on two legs. This frees our arms and hands for other functions such as carrying objects.
- Opposable thumbs – The thumb is positioned in the opposite direction of the other fingers. This allows humans to manipulate small objects.
- Large brain in relation to body size
- Capacity for complex language –Other species are able to vocalize sounds for mating, warnings or identification purposes only. Humans have the ability to produce complex sounds and languages and are able to communicate in written forms.
The Levels of Organization of the Human Body
The human body is organized according to several criteria from the smallest level (atoms) to the entire organism as you will read below.
To study the chemical level of organization, scientists consider the simplest building blocks of matter: subatomic particles, atoms and molecules. All matter in the universe is composed of one or more unique pure substances called elements, familiar examples of which are hydrogen, oxygen, carbon, nitrogen, calcium, and iron. The smallest unit of any of these pure substances (elements) is an atom. Atoms are made up of subatomic particles such as the proton, electron and neutron. Two or more atoms combine to form a molecule, such as the water molecules, proteins, and sugars found in living things. Molecules are the chemical building blocks of all body structures.
A cell is the smallest independently functioning unit of a living organism. Even bacteria, which are extremely small, independently living organisms, have a cellular structure. Each bacterium is a single cell. All living structures of human anatomy contain cells, and almost all functions of human physiology are performed in cells or are initiated by cells.
A human cell typically consists of flexible membranes that enclose cytoplasm, a water-based cellular fluid together with a variety of tiny functioning units called organelles. In humans, as in all organisms, cells perform all functions of life. A tissue is a group of many similar cells (though sometimes composed of a few related types) that work together to perform a specific function. An organ is an anatomically distinct structure of the body composed of two or more tissue types. Each organ performs one or more specific physiological functions. An organ system is a group of organs that work together to perform major functions or meet physiological needs of the body.
There are eleven distinct organ systems in the human body. Assigning organs to organ systems can be imprecise since organs that “belong” to one system can also have functions integral to another system. In fact, most organs contribute to more than one system. In this course, we will discuss some, but not all, of these organ systems.
Review
- What is biodiversity? Identify three ways that biodiversity may be measured.
- Define biological species. Why is this definition often difficult to apply?
- Explain why it is important to classify living things and outline the Linnaean system of classification.
- What is binomial nomenclature? Give an example.
- Contrast Linnaean and phylogenetic systems of classification.
- Describe the taxon called the domain, and compare the three widely recognized domains of living things.
- True or False. Humans have identified all of the species on Earth.
- True or False. In the binomial nomenclature for humans, Homo is the genus and sapiens refers to the specific species.
- What is the difference between Prokaryotes and Eukaryotes?
- What are the characteristics that set humans apart from other species on Earth?
1.3 What is Science?
This individual in Figure 1.7 is getting a flu vaccine. You probably know that getting a vaccine can hurt, but it’s usually worth it. A vaccine contains dead or altered forms of “germs” that normally cause a disease, such as flu or measles. The germs in vaccines have been inactivated or weakened so they can no longer cause illness, but they are still “noticed” by the immune system. They stimulate the immune system to produce chemicals that can kill the actual germs if they enter the body, thus preventing future disease. How was such an ingenious way to prevent disease discovered? The short answer is more than two centuries of science.
Figure 1.7: Getting an annual flu shot
Science as Process
You may think of science as a large and detailed body of knowledge, but science is actually more of a process than a set of facts. The real focus of science is the accumulation and revision of scientific knowledge. Science is a special way of gaining knowledge that relies on evidence and logic. Evidence is used to continuously test ideas. Through time, with repeated evidence gathering and testing, scientific knowledge advances.
We’ve been accumulating knowledge of vaccines for more than two centuries. The discovery of the first vaccine, as well as the process of vaccination, dates back to 1796. An English doctor named Edward Jenner observed that people who became infected with cowpox did not get sick from smallpox, a similar but much more virulent disease (Figure 1.8). Jenner decided to transmit cowpox to a young child to see if it would protect them from smallpox. He gave the child cowpox by scratching liquid from cowpox sores into the child’s skin. Then, six weeks later, he scratched liquid from smallpox sores into the child’s skin. As Jenner predicted, the child did not get sick from smallpox. Jenner had discovered the first vaccine, although additional testing was needed to show that it really was effective.
Figure 1.8: A young child covered with skin lesions from smallpox. Until it was eradicated, this highly contagious infection caused many deaths, and those that survived were often severely scarred for life.
Almost a century passed before the next vaccine was discovered, a vaccine for cholera in 1879. Around the same time, French chemist Louis Pasteur found convincing evidence that many human diseases are caused by germs. This earned Pasteur the title of “father of germ theory.” Since Pasteur’s time, vaccines have been discovered for scores of additional diseases caused by “germs,” and scientists are currently researching vaccines for many others.
Benefits of Science
Medical advances such as the discovery of vaccines are one of the most important benefits of science, but science and scientific knowledge are also crucial for most other human endeavors. Science is needed to design safe cars, predict storms, control global warming, develop new technologies of many kinds, help couples have children, and put humans on the moon! Clearly, the diversity of applications of scientific knowledge is vast!
Review
- Explain why science is more accurately considered a process than a body of knowledge.
- Jenner used a young boy as a research subject in his smallpox vaccine research. Today, scientists must follow strict guidelines when using human subjects in their research. What unique concerns do you think might arise when human beings are used as research subjects?
- What gave Jenner the idea to develop a vaccine for smallpox?
- Why do you think almost a century passed between the development of the first vaccine (for smallpox) and the development of the next vaccine (for cholera)?
- How does science influence your daily life?
Explore More
Check out this video to learn more about the smallpox vaccine:
Defining Science
Science is a distinctive way of gaining knowledge about the natural world that starts with a question and then tries to answer the question with evidence and logic. Science is an exciting exploration of all the whys and hows that any curious person might have about the world. You can be part of that exploration. Besides your curiosity, all you need is a basic understanding of how scientists think and how science is done.
Thinking Like a Scientist
Thinking like a scientist rests on certain underlying assumptions. Scientists assume that:
- Nature can be understood through systematic study.
- Scientific ideas are open to revision.
- Sound scientific ideas withstand the test of time.
- Science cannot provide answers to all questions.
Nature is Understandable
Scientists think of nature as a single system controlled by natural laws. By discovering natural laws, scientists strive to increase their understanding of the natural world. Laws of nature are expressed as scientific laws. A scientific law is a statement that describes what always happens under certain conditions in nature.
Examples of scientific laws include Mendel’s Laws of Inheritance. These laws were discovered by an Austrian Monk, named Gregor Mendel (Figure 1.9), in the mid-1800s. The laws describe how certain traits are inherited from parents by their offspring. Although Mendel discovered his laws of inheritance by experimenting with pea plants, we now know that the laws apply to many other organisms, including human beings. The laws describe how we inherit relatively simple genetic traits, such as blood type, from our parents. For example, if you know the blood types of your parents, you can use Mendel’s laws to predict your chances of having a particular blood type.
Barbara McClintock (Figure 1.9) added to our understanding of inheritance in the 1950s by discovering how chromosomes exchange information during meiosis. Meiosis is how organisms produce reproductive cells (such as egg or sperm). McClintock worked with corn and, using the color traits in the kernels demonstrated how crossing-over is used to exchange information between chromosomes. An understanding of how crossing-over works is essential to our understanding of inheritance because it explains why using Mendelian rules of inheritance does not always produce the correct ratios.
Figure 1.9: Science is an ongoing process of gaining knowledge. Gregor Mendel discovered laws of inheritance in the mid-1800s. Barbara McClintock refined these laws in the 1950s. Many other scientists have also contributed to our understanding of inheritance.
Scientific Ideas are Open to Change
Science is more of a process than a set body of knowledge. Scientists are always testing and revising their ideas, and as new observations are made, existing ideas may be challenged. Ideas may be replaced with new ideas that better fit the facts, but more often existing ideas are simply revised. For example, when scientists discovered how genes control genetic traits, they didn’t throw out Mendel’s laws of inheritance. The new discoveries helped to explain why Mendel’s laws applied to certain traits but not others. They showed that Mendel’s laws are part of a bigger picture. Through many new discoveries over time, scientists gradually build an increasingly accurate and detailed understanding of the natural world.
Occasionally, scientific ideas change radically. Radical changes in scientific ideas were given the name paradigm shifts by the philosopher Thomas Kuhn in 1962. Kuhn agreed that scientific knowledge typically accumulates gradually, as new details are added to established theories. However, Kuhn also argued that from time to time, a scientific revolution occurs in which current theories are abandoned and completely new ideas take their place.
Although there is debate among scientists as to what constitutes a paradigm shift, the theory of evolution is widely accepted as a good example in biology. In fact, some scientists argue that it is the only example of a paradigm shift in biology. Prior to Charles Darwin’s publication of his theory of evolution in the 1860s, most scientists believed that God had created living species and that the species on Earth had not changed since they were created. Drawing on a great deal of evidence and logical arguments, Darwin demonstrated that species could change and that new species could arise from pre-existing ones. This was such a radical change in scientific thinking that Darwin was reluctant to publish his ideas for fear of a backlash from other scientists and the public. Indeed, Darwin was at first ridiculed for his evolutionary theory, but in time, it was widely accepted and became a cornerstone of all life sciences.
Scientific Knowledge May Be Long Lasting
Many scientific ideas have withstood the test of time. For example, about 200 years ago, the scientist John Dalton proposed atomic theory — the theory that all matter is made of tiny particles called atoms. This theory is still valid today. During the two centuries since the theory was first proposed, a great deal more has been learned about atoms and the even smaller particles of which they are composed. Nonetheless, the idea that all matter consists of atoms remains valid. There are many other examples of basic scientific ideas that have been tested repeatedly and found to be sound. You will learn about many of them as you study human biology.
Not All Questions Can be Answered by Science
Science rests on evidence and logic, and evidence comes from observations. Therefore, science deals only with things that can be observed. An observation is anything that is detected through human senses or with instruments and measuring devices that extend human senses. Things that cannot be observed or measured by current means — such as supernatural beings or events — are outside the bounds of science. Consider these two questions about life on Earth:
- Did life on Earth evolve over time?
- Was life on Earth created by a supernatural deity?
The first question can be answered by science on the basis of scientific evidence such as fossils and logical arguments. The second question could be a matter of belief but no evidence can be gathered to support or refute it. Therefore, it is outside the realm of science.
Feature: Human Biology in the News
Scientific research is often reported in the popular media. In fact, that’s how most people learn about new scientific findings. Informing the public about scientific research is a valuable media service, but the types of scientific investigations that are reported may lead to a distorted public perception of what science is and how reliable its results are. Why? There are actually two types of science, often referred to as consensus science and frontier science. The latter type of science is the type that usually makes the news, but the media generally do not distinguish between the two types. Therefore, many people may infer that what they read about frontier science is typical of all science.
- Consensus science refers to scientific ideas that have been researched for a long period of time and for which a great deal of evidence has accumulated. This type of research generally fits well within current scientific paradigms. A good example of consensus science is global climate change. Data showing the impact of increasing levels of atmospheric carbon dioxide, due to human activities, on global warming have been accumulating for many decades. Today, virtually all climate scientists agree that global warming is occurring and that human actions are largely responsible for it. However, the few scientists — and many politicians — who do not agree with the consensus view receive greater media attention because the consensus view is “old” news. The findings have been coming in for years, and new research in the area keeps finding similar results.
- Frontier science, in contrast, refers to scientific ideas that are relatively new and have not yet been supported by years of scientific evidence. Frontier research takes place at the frontiers of knowledge in a particular field. A good example of frontier science is research into the presumed link between cholesterol in the diet and cholesterol in the blood. The consensus view for many years was that a diet high in cholesterol increases blood levels of cholesterol, which may lead, in turn, to cardiovascular disease. Recent research challenging this accepted view found that genes play a more significant role than diet in blood levels of cholesterol and risk of cardiovascular disease.
The media tend to focus on frontier science because it seems controversial and may lead to major new scientific breakthroughs. With more research, ideas in frontier science may be supported by more evidence, gain wider acceptance, and become consensus science. In some cases, frontier science that is at odds with a current paradigm may even lead to a paradigm shift. However, the opposite may happen instead. Additional research may undermine the initial findings of frontier research so that the new and exciting ideas are rejected. Unfortunately, when frontier science is later shown to be mistaken, people may infer that all science, including consensus science, is unreliable.
1.4: Theories in Science
What Is a Scientific Theory?
A scientific theory is a broad explanation of events that is widely accepted by the scientific community. To become a theory, an explanation must be strongly supported by a great deal of evidence.
People commonly use the word theory to describe a guess or hunch about how or why something happens. For example, you might say, “I think a woodchuck dug this hole in the ground, but it’s just a theory.” Using the word theory in this way is different from the way it is used in science. A scientific theory is not just a guess or hunch that may or may not be true. In science, a theory is an explanation that has a high likelihood of being correct because it is so well supported by evidence.
How does a scientific theory differ from a scientific law? Watch this TED animation to find out.
1.5: Scientific Investigations
If you were walking in the woods and saw this stream, you probably would wonder what made the water turn orange. Is the water orange because of something growing in it? Is it polluted with some kind of chemicals? To answer these questions, you might do a little research. For example, you might ask local people if they know why the water is orange, or you might try to learn more about it online. If you still haven’t found answers, you could undertake a scientific investigation. In short, you could “do” science.
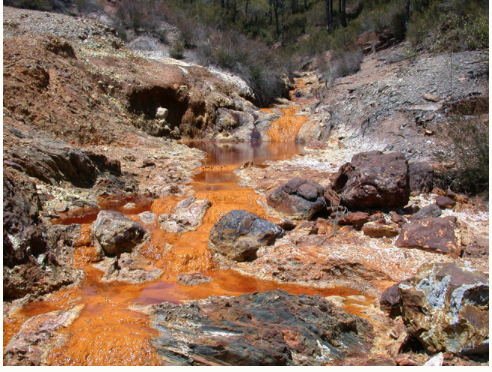
Science is more about doing than knowing. Scientists are always trying to learn more and gain a better understanding of the natural world. There are basic methods of gaining knowledge that is common to all of science. At the heart of science is the scientific investigation. A scientific investigation is a plan for asking questions and testing possible answers in order to advance scientific knowledge. Figure 1.12 outlines the steps of the scientific method. Science textbooks often present this simple, linear “recipe” for a scientific investigation. This is an oversimplification of how science is actually done, but it does highlight the basic plan and purpose of any scientific investigation: testing ideas with evidence. We will use this flowchart to help explain the overall format for scientific inquiry.
Science is actually a complex endeavor that cannot be reduced to a single, linear sequence of steps, like the instructions on a package of cake mix. Real science is nonlinear, iterative (repetitive), creative, unpredictable, and exciting. Scientists often undertake the steps of an investigation in a different sequence, or they repeat the same steps many times as they gain more information and develop new ideas. Scientific investigations often raise new questions as old ones are answered. Successive investigations may address the same questions but at ever-deeper levels. Alternatively, an investigation might lead to an unexpected observation that sparks a new question and takes the research in a completely different direction.
Knowing how scientists “do” science can help you in your everyday life, even if you aren’t a scientist. Some steps of the scientific process — such as asking questions and evaluating evidence — can be applied to answering real-life questions and solving practical problems.
Figure 1.11: The Scientific Method: The scientific method is a process for gathering data and processing information. It provides well-defined steps to standardize how scientific knowledge is gathered through a logical, rational problem-solving method. This diagram shows the steps of the scientific method, which are listed below.
A scientific investigation typically begins with observations. An observation is anything that is detected through human senses or with instruments and measuring devices that enhance human senses. We usually think of observations as things we see with our eyes, but we can also make observations with our sense of touch, smell, taste, or hearing. In addition, we can extend and improve our own senses with instruments such as thermometers and microscopes. Other instruments can be used to sense things that human senses cannot detect at all, such as ultraviolet light or radio waves.
Sometimes chance observations lead to important scientific discoveries. One such observation was made by the Scottish biologist Alexander Fleming (Figure 1.12) in the 1920s. Fleming’s name may sound familiar to you because he is famous for the discovery in question. Fleming had been growing a certain type of bacteria on glass plates in his lab when he noticed that one of the plates had been contaminated with mold. On closer examination, Fleming observed that the area around the mold was free of bacteria.
Figure 1.12: Alexander Fleming experimenting with penicillin and bacteria in his lab in the 1940s.
Asking Questions
Observations often lead to interesting questions. This is especially true if the observer is thinking like a scientist. Having scientific training and knowledge is also useful. Relevant background knowledge and logical thinking help make sense of observations so the observer can form particularly salient questions. Fleming, for example, wondered whether the mold — or some substance it produced — had killed bacteria on the plate. Fortunately for us, Fleming didn’t just throw out the mold-contaminated plate. Instead, he investigated his question and in so doing, discovered the antibiotic penicillin.
Hypothesis Formation
To find the answer to a question, the next step in a scientific investigation typically is to form a hypothesis. A hypothesis is a possible answer to a scientific question. But it isn’t just any answer. A hypothesis must be based on scientific knowledge. In other words, it shouldn’t be at odds with what is already known about the natural world. A hypothesis also must be logical, and it is beneficial if the hypothesis is relatively simple. In addition, to be useful in science, a hypothesis must be testable and falsifiable. In other words, it must be possible to subject the hypothesis to a test that generates evidence for or against it, and it must be possible to make observations that would disprove the hypothesis if it really is false.
A hypothesis is often expressed in the form of prediction: If the hypothesis is true, then B will happen to the dependent variable. Fleming’s hypothesis might have been: “If a certain type of mold is introduced to a particular kind of bacteria growing on a plate, the bacteria will die.” Is this a good and useful hypothesis? The hypothesis is logical and based directly on observations. The hypothesis is also simple, involving just one type each of mold and bacteria growing on a glass plate. This makes it easy to test. In addition, the hypothesis is falsifiable. If bacteria were to grow in the presence of the mold, it would disprove the hypothesis if it really is false.
Hypothesis Testing
Hypothesis testing is at the heart of a scientific investigation. How would Fleming test his hypothesis? He would gather relevant data as evidence. Evidence is any type of data that may be used to test a hypothesis. Data (singular, datum) are essentially just observations. The observations may be measurements in an experiment or just something the researcher notices. Testing a hypothesis then involves using the data to answer two basic questions:
- If my hypothesis is true, what would I expect to observe?
- Does what I actually observe match what predicted?
A hypothesis is supported if the actual observations (data) match the expected observations. A hypothesis is refuted if the actual observations differ from the expected observations.
Testing Fleming’s Hypothesis
To test his hypothesis that the mold kills bacteria, Fleming grew colonies of bacteria on several glass plates and introduced mold to just some of the plates. He subjected all of the plates to the same conditions except for the introduction of mold. Any differences in the growth of bacteria on the two groups of plates could then be reasonably attributed to the presence/absence of mold. Fleming’s data might have included actual measurements of bacterial colony size, like the data shown in the data table below, or they might have been just an indication of the presence or absence of bacteria growing near the mold. Data like the former, which can be expressed numerically, are called quantitative data. Data like the latter, which can only be expressed in words, such as present or absent, are called qualitative data.
What Is an Experiment?
An experiment is a special type of scientific investigation that is performed under controlled conditions. Like all investigations, an experiment generates evidence to test a hypothesis. But unlike some other types of investigations, an experiment involves manipulating some factors in a system in order to see how it affects the outcome. Ideally, experiments also involve controlling as many other factors as possible in order to isolate the cause of the experimental results.
An experiment generally tests how one particular variable is affected by some other specific variable. The affected variable is called the dependent variable or outcome variable. The variable that affects the dependent variable is called the independent variable. It is also called the manipulated variable because this is the variable that is manipulated by the researcher. Any other variables (control variable) that might also affect the dependent variable are held constant, so the effects of the independent variable alone are measured.
The spots on this child’s tongue are an early sign of vitamin C deficiency, which is also called scurvy. This disorder, which may be fatal, is uncommon today because foods high in vitamin C are readily available. They include tomatoes, peppers, and citrus fruits such as oranges, lemons, and limes. However, scurvy was a well-known problem on navy ships in the 1700s. It was said that scurvy caused more deaths in the British fleet than French and Spanish arms. At that time, the cause of scurvy was unknown and vitamins had not yet been discovered. Anecdotal evidence suggested that eating citrus fruits might cure scurvy. However, no one knew for certain until 1747, when a Scottish naval physician named John Lind did an experiment to test the idea. Lind’s experiment was one of the first clinical experiments in the history of medicine.
.jpg?revision=1&size=bestfit&width=209&height=195)
Figure 1.13: Scorbutic tongue
Lind’s Scurvy Experiment
Lind began his scurvy experiment onboard a British ship after it had been at sea for two months and sailors had started showing signs of scurvy. He chose a group of 12 sailors with scurvy and divided the group into 6 pairs. All 12 sailors received the same diet, but each pair also received a different daily supplement to the diet (Table 1)
Table 1: Lind’s Scurvy Experiment
Pair of Subjects | Daily Supplement to the Diet Received by this Pair |
1 | 1 quart of cider |
2 | 5 drops of sulfuric acid |
3 | 6 spoons of vinegar |
4 | 1 cup of seawater |
5 | 2 oranges and 1 lemon |
6 | spicy paste and a drink of barley water |
Lind’s experiment ended after just five days when the fresh citrus fruits ran out for pair 5. However, the two sailors in this pair had already fully recovered or greatly improved. The sailors in pair 1 (receiving the quart of cider) also showed some improvement, but sailors in the other pairs showed none.
Can you identify the independent and dependent variables in Lind’s experiment? The independent variable is the daily supplement received by the pairs. The dependent variable is the improvement/no improvement in scurvy symptoms. Lind’s results supported the citrus fruit cure for scurvy, and it was soon adopted by the British navy with good results. However, the fact that scurvy is caused by a vitamin C deficiency was not discovered until almost 200 years later.
Sampling
Lind’s scurvy experiment included just 12 subjects. This is a very small sample by modern scientific standards. The sample in an experiment or other investigation consists of the individuals or events that are actually studied. It rarely includes the entire population because doing so would likely be impractical or even impossible.
There are two types of errors that may occur by studying a sample instead of the entire population: chance error and bias.
- A chance error occurs if the sample is too small. The smaller the sample is, the greater the chance that it does not fairly represent the whole population. Chance error is mitigated by using a larger sample.
- Bias occurs if the sample is not selected randomly with respect to a variable in the study. This problem is mitigated by taking care to choose a randomized sample.
A reliable experiment must be designed to minimize both of these potential sources of error. You can see how the sources of error were addressed in another landmark experiment: Jonas Salk’s famous 1953 trial of his newly developed polio vaccine. Salk’s massive experiment has been called the “greatest public health experiment in history.”
Salk’s Polio Vaccine Experiment
Imagine a nationwide epidemic of a contagious flu-like illness that attacks mainly children and often causes paralysis. That’s exactly what happened in the U.S. during the first half of the 20th century. Starting in the early 1900s, there were repeated cycles of polio epidemics, and each seemed to be stronger than the one before. Many children ended up on life support in so-called “iron lungs” (see photo below) because their breathing muscles were paralyzed by the disease.
Polio is caused by a virus, and there is still no cure for this potentially devastating illness. Fortunately, it can now be prevented with vaccines. The first polio vaccine was discovered by Jonas Salk in 1952. After testing the vaccine on himself and his family members to assess its safety, Salk undertook a nationwide experiment to test the effectiveness of the vaccine using more than a million schoolchildren as subjects. It’s hard to imagine a nationwide trial of an experimental vaccine using children as “guinea pigs.” It would never happen today. However, in 1953, polio struck such fear in the hearts of parents that they accepted Salk’s word that the vaccine was safe and gladly permitted their children to participate in the study.
Salk’s experiment was very well designed. First, it included two very large, random samples of children — 600,000 in the treatment group, called the experimental group, and 600,000 in the untreated group, called the control group. Using very large and randomized samples reduced the potential for chance error and bias in the experiment. Children in the experimental group were injected with the experimental polio vaccine. Children in the control group were injected with a harmless saline (saltwater) solution. The saline injection was a placebo. A placebo is a “fake” treatment that actually has no effect on health. It is included in trials of vaccines and other medical treatments, so subjects will not know in which group (control or experimental) they have been placed. The use of a placebo helps researchers control for the placebo effect. This is a psychologically-based reaction to a treatment that occurs just because the subject is treated, even if the treatment has no real effect.
Experiments in which a placebo is used are generally blind experiments because the subjects are “blind” to their experimental group. This helps prevent bias in the experiment. Often, even the researchers do not know which subjects are in each group. This type of experiment is called a double-blind experiment because both subjects and researchers are “blind” to which subjects are in each group. Salk’s vaccine trial was a double-blind experiment, and double-blind experiments are now considered the gold standard of clinical trials of vaccines, therapeutic drugs, and other medical treatments.
Salk’s polio vaccine proved to be highly successful. Analysis of data from his study revealed that the vaccine was 80 to 90 percent effective in preventing polio. Almost overnight, Salk was hailed as a national hero. He appeared on the cover of Time magazine and was invited to the White House. Within a few years, millions of children had received the polio vaccine. By 1961, the incidence of polio in the U.S. had been reduced by 96 percent.
Limits on Experimentation
Well-done experiments are generally the most rigorous and reliable scientific investigations. However, their hallmark feature of manipulating variables to test outcomes is not possible, practical, or ethical in all investigations. As a result, many ideas cannot be tested through experimentation. For example, experiments cannot be used to test ideas about what our ancestors ate millions of years ago or how long-term cigarette smoking contributes to lung cancer. In the case of our ancestors, it is impossible to study them directly. Researchers must rely instead on indirect evidence, such as detailed observations of their fossilized teeth. In the case of smoking, it is unethical to expose human subjects to harmful cigarette smoke. Instead, researchers may use large observational studies of people who are already smokers, with nonsmokers as controls, to look for correlations between smoking habits and lung cancer.
Review
- Identify the independent and dependent variables in Salk’s nationwide polio vaccine trial.
- What is the placebo effect? Explain how Salk’s experimental design controlled for it.
- Fill in the blanks. The _____________ variable is manipulated to see the effects on the ___________ variable.
- True or False. Experiments cannot be done on humans.
- True or False. Larger sample sizes are generally better than smaller ones in scientific experiments.
- Answer the following questions about Lind’s scurvy experiment.
- Why do you think it was important that the sailors’ diets were all kept the same, other than the daily supplement?
- Can you think of some factors other than diet that could have potentially been different between the sailors that might have affected the outcome of the experiment?
- Why do you think the sailors who drank cider had some improvement in their scurvy symptoms?
- Explain why double-blind experiments are considered to be more rigorous than regular blind experiments.
1.8: Case Study Shot – Why Should You Learn About Science?
Case Study: To Give a Shot or Not
After reading the chapter and case study, think about the following questions:
- What do you think about the quality of Elena’s online source of information about vaccines compared to Dr. Rodriguez’s sources?
- Do you think the arguments presented here that claim that the MMR vaccine causes autism are scientifically valid? Could there be alternative explanations for the observations?
- Why do you think diseases like measles, polio, and mumps are rare these days, and why are we still vaccinating for these diseases?
New mother Elena left her pediatrician’s office still unsure whether to vaccinate baby Juan. Dr. Rodriguez gave Elena a list of reputable sources where she could look up information about the safety of vaccines herself, such as the Centers for Disease Control and Prevention (CDC). Elena reads that the consensus within the scientific community is that there is no link between vaccines and autism. She finds a long list of studies published in peer-reviewed scientific journals that disprove any link. Additionally, some of the studies are “meta-analyses” that analyzed the findings from many individual studies. Elena is reassured by the fact that many different researchers, using a large number of subjects in numerous well-controlled and well-reviewed studies, all came to the same conclusion.
Elena also went back to the author’s website that originally scared her about the safety of vaccines. She found that the author was not a medical doctor or scientific researcher, but rather was a self-proclaimed “child wellness expert.” Also, the doctor sold books and advertising on their site, some of which were related to claims of vaccine injury. Elena realized that the doctor was both an unqualified and potentially biased source of information.
Also, Elena realized that some of the doctor’s arguments were based on correlations between autism and vaccines, but, as the saying goes, “correlation does not imply causation.” For instance, the recent rise in autism rates may have occurred during the same time period as an increase in the number of vaccines given in childhood, but Elena could think of many other environmental and social factors that have also changed during this time period. There are just too many variables to come to the conclusion that vaccines, or anything else, are the cause of the rise in autism rates based on that type of argument alone. Also, Elena learned that the age of onset of autism symptoms happens to typically be around the time that the MMR vaccine is first given, so the apparent association in the timing may just be a coincidence.
Public health, sanitation, and the use of antibiotics and vaccines have lessened the impact of infectious disease on human populations. Through vaccination programs, better nutrition, and vector control (carriers of disease), international agencies have significantly reduced the global infectious disease burden. Reported cases of measles in the United States dropped from around 700,000 a year in the 1950s to practically zero by the late 1990s and declared eradicated by the year 2000 (Figure 1.14). Globally, measles fell 60 percent from an estimated 873,000 deaths in 1999 to 164,000 in 2008. This advance is attributed entirely to a comprehensive vaccination program.
Figure 1.15: Measles cases reported in the United States, 1944-2007. From 1944 to 1963 measles cases fluctuated between 100 – 800 cases per thousand individuals. The measles vaccine was licensed in 1963 and the number of cases plummeted to less than 100 per thousand individuals. A second dose was recommended in 1988 an causes the total number of cases to fall to nearly zero.
However, Elena came across news about a measles outbreak that originated in California in 2014, 2015, and the latest outbreak of 2019 (Figure 1.16). Measles wasn’t just a disease of the past as she had thought! She learned that measles and whooping cough, which had previously been rare thanks to widespread vaccinations, are now on the rise, and that people choosing not to vaccinate their children seems to be one of the contributing factors. Elena realized that it is important to vaccinate their baby against these diseases, not only to protect the baby from their potentially deadly effects but to also protect others in the population.
In her reading, Elena learns that scientists do not yet know the causes of autism, but she feels reassured by the abundance of data that disproves any link with vaccines. She thinks that the potential benefit of protecting their baby’s health against deadly diseases outweighs any unsubstantiated claims about vaccines. She will be making an appointment to get baby Juan their shots soon.
Figure 1.16: Measles cases reported in the US as of June 2019 – 1044 cases of measles were reported in the states of Arizona, California, Colorado, Connecticut, Florida, Georgia, Idaho, Illinois, Indiana, Iowa, Kentucky, Maine, Maryland, Massachusetts, Michigan, Missouri, New Mexico, Nevada, New Hampshire, New Jersey, New York, Oklahoma, Oregon, Pennsylvania, Texas, Tennessee, Virginia, and Washington. This is much higher than any year in the past decade where the number of cases fluctuated from 55 to 667.
Chapter Summary Review
- Which of the following is the best example of “doing science?”
- memorizing the processes of the water cycle
- learning how to identify trees from their leaves
- learning the names of all the bones in the human body
- making observations of wildlife while hiking in the woods
- A scientist develops a new idea based on their observations of nature. What should they do next?
- think of a way to test the idea
- claim that they have discovered a new theory
- reject any evidence that conflicts with the idea
- look only for evidence that supports the idea
- Which of the following is defined as a possible answer to a scientific question?
- an observation
- data
- a hypothesis
- statistics
- Do scientists usually come up with a hypothesis in the absence of any observations? Explain your answer.
- Why does a good hypothesis have to be falsifiable?
- Name one scientific law.
- Name one scientific theory.
- Give an example of a scientific idea that was later discredited.
- Would the idea that the Earth revolves around the Sun be considered consensus science or frontier science?
- True or False. A scientific investigation always follows the same sequence of steps in a linear fashion.
- True or False. Data that does not support a hypothesis is not useful.
- True or False. Experimentation is the only valid type of scientific investigation.
- Why is it important that scientists communicate their findings to others? How do they usually do this?
- What is a “control group” in science?
- In a scientific experiment, why is it important to only change one variable at a time?
- Which is the dependent variable – the variable that is manipulated or the variable that is being affected by the change?
- You see an ad for a “miracle supplement” called NQP3 that claims the supplement will reduce belly fat. They say it works by reducing the hormone cortisol and by providing your body with missing unspecified “nutrients”, but they do not cite any peer-reviewed clinical studies. They show photographs of three people who appear slimmer after taking the product. A board-certified plastic surgeon endorses the product on television. Answer the following questions about this product.
- Do you think that because a doctor endorsed the product, it really works? Explain your answer.
- Do you think the photographs are good evidence that the product works? Why or why not?
Attributions
This chapter is composed of text taken from of the following sources:
Wakim, Suzanne, and Mandeep Grewal. Human Biology. Creative Commons Attribution 4.0 International License, 2024. [Retrieved from Human Biology (Wakim & Grewal) – Biology LibreTexts]
Cushwa, W., & Senior Contributors. (2015). Human biology. OpenStax CNX. [CC BY 4.0 license]. [Retrieved from Human Biology : Willy Cushwa : Free Download, Borrow, and Streaming : Internet Archive]